Stay Up to Date
Submit your email address to receive the latest industry and Aerospace America news.
The CFD Vision 2030 Integration Committee advocates for, inspires and enables community activities recommended by the vision study for revolutionary advances in the state-of-the-art of computational technologies needed for analysis, design and certification of future aerospace systems.
At the fourth High Lift Prediction Workshop, or HLPW4, in January, multiple organizations accurately predicted maximum lift coefficient values for a complex high-lift aircraft configuration. The predictions were based on scale resolving methods including Wall Modeled Large Eddy Simulation, or WMLES, and Hybrid Reynolds Averaged Navier-Stokes/Large Eddy Simulation methods running on meshes ranging from 100 million to 2 billion spatial degrees of freedom. These simulations were compared to test results of the Common Research Model-High Lift configuration tested in QinetiQ’s 5-meter wind tunnel in the U.K. These comparisons demonstrated the importance of including the geometric details of the wind tunnel in the simulations. Additionally, solution-based adaptive approaches from multiple groups demonstrated results that were in strong agreement with each other in grid-converged limits. Government, industry and commercial codes produced consistent maximum lift coefficient values results showing broader adoption of these advanced techniques. These results demonstrate significant progress toward the CFD Vision 2030 Roadmap’s goal of achieving “unsteady, complex geometry, separated flow predictions at flight Reynolds numbers.”
At a June seminar, the Launch, Ascent, and Vehicle Aerodynamics group at NASA’s Ames Research Center in California presented results from its WMLES in a Cartesian immersed boundary framework on a high lift configuration. These results demonstrated that mesh generation time can be reduced by over two orders of magnitude, or about two months for the structured-overset case to a few hours, by using the immersed boundary approach. The accuracy of aerodynamics predictions was maintained on the same case that was used during HLPW4. This work combines a scale resolving solver with an automated mesh generator — key elements of the roadmap — to produce accurate solutions with a large reduction in time required to go from geometry to solution.
Steps toward demonstrating consistent results across multiple solvers were also demonstrated at the first High-Fidelity CFD Verification Workshop following AIAA’s SciTech forum in January. A mesh-motion test suite demonstrated three to five digits of precision and the shock location showed agreement to within four to five digits of precision for a steady supersonic case across multiple participants. A test suite for verification of the Spalart-Allmaras One-Equation Model with Quadratic Constitutive Relation, 2000, or SA-QCR2000, turbulence model demonstrated excellent mesh convergence and agreement between participants for 2D solutions, but 3D mesh convergence without solution-based adaptation remains challenging. Significant challenges were also identified in trying to demonstrate agreement with a smooth-body separation test case using wall-modeled large eddy simulations. These challenges highlight continued research needs in algorithms, meshing and modeling to meet Vision 2030 objectives.
Prediction of entry capsule dynamic stability, particularly in the transonic flight regime, is challenging the RANS state-of-the-art. NASA engineers throughout the year used forced oscillation and free-flight CFD techniques to study this problem and evaluate the impact of application of scale-resolving methods to the simulation of the massively separated dynamic wakes behind these vehicles. This year’s assessments of these methods on the Mars Sample Return Earth Entry System, the Dragonfly Titan Entry and Descent System and commercial space vehicles showed that inclusion of nonlinear separated flow wake effects can affect the aerodynamic damping of these vehicles during transition from low supersonic, through transonic, to subsonic flight.
Stay Up to Date
Submit your email address to receive the latest industry and Aerospace America news.
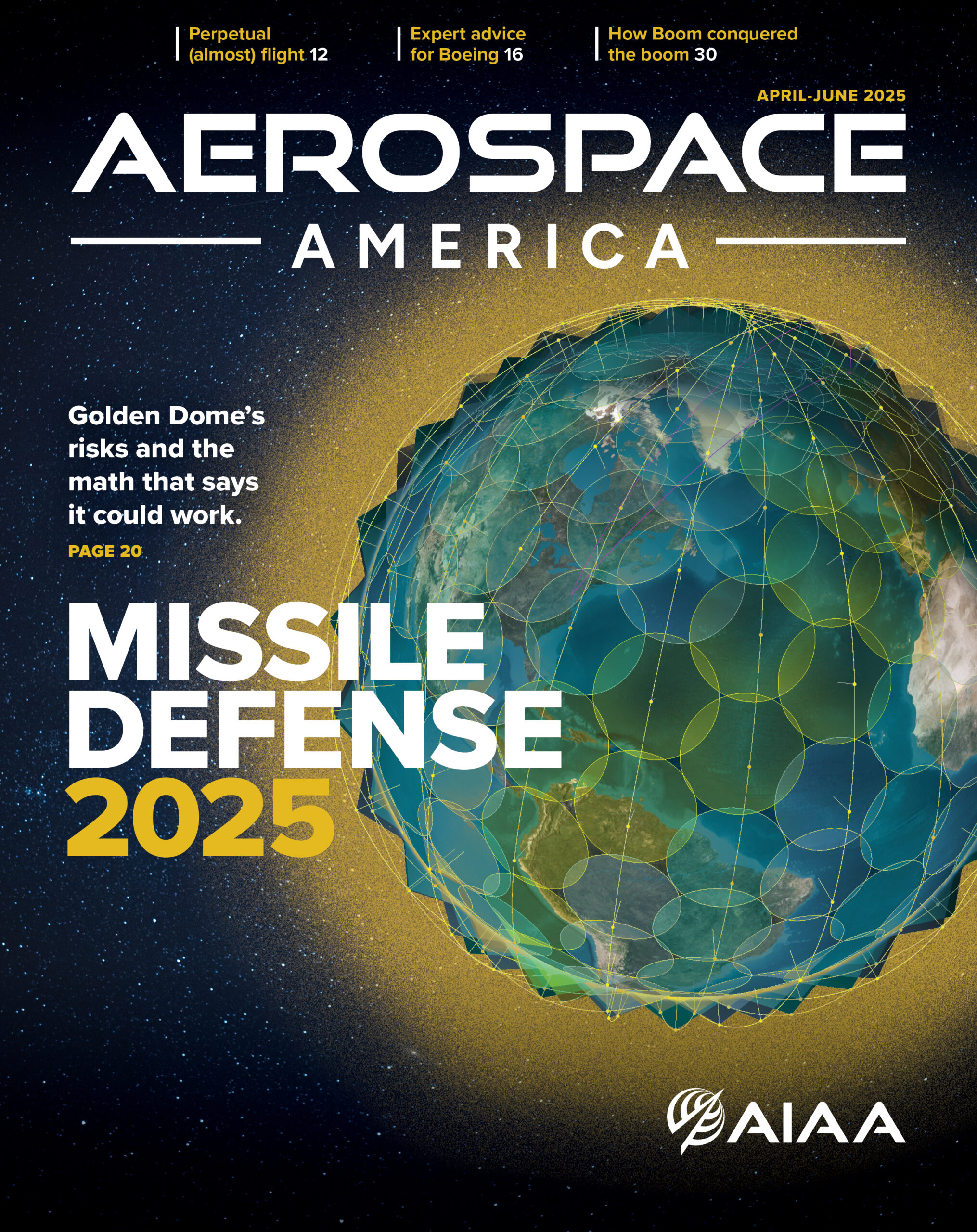