Stay Up to Date
Submit your email address to receive the latest industry and Aerospace America news.
Precise navigation will be a necessity for safe human exploration of Mars and other celestial bodies in deep space. A pair of experiments about to get underway could change the way this navigation is done, and for the better.
Making coffee and whatnot, future humans traversing the solar system move around inside their tiny tin can — I mean spaceship — throwing off the trajectory little by little. But this drift is no biggie. By this time, space navigators back on Earth no longer have to spend hours discovering a slight shift in trajectory by sending radio waves to the spacecraft from NASA’s Deep Space Network antennas on three continents, waiting for return signals, and calculating the distance to the spacecraft and its position.
Instead, sensors onboard our notional spaceship-of-the-future harness the quantum properties of atoms to detect the drift, needing only a reference signal from Earth plus an onboard atomic clock to do it. The spaceship’s flight computer automatically corrects the course in real time.
A quantum-driven navigation revolution like this could reduce the risks of deadly mistakes, such as a failed atmospheric entry or a misdirected thruster firing, during a human mission to Mars in the 2030s. Physicists from NASA and several universities are working with industry researchers to perfect the necessary science and technology.
Specifically, an experimental Deep Space Atomic Clock, built in California at the NASA-funded Jet Propulsion Laboratory, is scheduled for launch in November as an instrument aboard a scientific satellite. Not to be outdone, university physicists are planning to run navigation-related quantum experiments inside an ice-chest-sized container aboard the International Space Station. This Cold Atom Laboratory, which the physicists will command remotely from JPL, remains in its calibration phase after astronauts set it up in May.
These projects were inspired in part by a 2011 report from the U.S. National Academy of Sciences, “Recapturing a Future for Space Exploration, Life and Physical Sciences Research for a New Era.” This decadal survey of spending priorities listed unlocking the potential of “quantum gases” — atoms that are so cold that their quantum properties become apparent — along with precision measurements, such as those made by atomic clocks, as two of four top recommended priorities for physical science in space.
I talked to some of the scientists and engineers helping to ensure that the first Earthlings exploring Mars or elsewhere beyond the moon won’t end up adrift.
Timing is everything
For a space mission to succeed, engine burns and other maneuvers must be timed precisely, and that’s not just true for missions with astronauts playing ping-pong and perturbing things. Consider NASA’s $800 million InSight lander now on its way to Mars for a planned arrival in November to begin a geology mission.
InSight, just as any spacecraft, experiences unpredictable small forces that can add up to trouble over millions of kilometers, forces such as inconsistencies in its thruster firings or pressure from the sun so slight that it’s undetectable to today’s inertial navigation systems, such as those in aircraft and the atmospheric landing systems of spacecraft.
Today, NASA tracks deep space spacecraft from the Deep Space Network stations in California, Spain and Australia — whichever station’s antennas have a direct line of sight to the spacecraft as Earth rotates. An atomic clock at each DSN station times how long the return signal takes to arrive, and navigators then calculate the spacecraft’s trajectory based partly on those transit times. They also factor in the frequency of the signal’s Doppler shift plus information from a third method, delta differential one-way ranging. In this technique, two antennas at a time receive signals from the spacecraft while also referencing radio waves from the same star-like quasar.
The process soaks up more of the DSN time than NASA would like. Each station must communicate with just one spacecraft at a time and, depending on where Earth and Mars are in their orbits around the sun, a signal can take 21 minutes to reach Earth from Mars or vice versa. Factor in computing and mission planning, and the whole process of plotting a spacecraft’s trajectory can take hours.
InSight, for instance, has been speeding along toward Mars in a relative tracking slumber, with NASA checking up on it one to three times a week throughout the “cruise” phase of the six-month trip. The spacecraft was poised to enter its 60-day approach subphase, which will require intensive tracking by one DSN ground station after another. Trajectory correction maneuvers were scheduled for four dates in October and November.
JPL space navigator Todd Ely, an aerospace engineer, wants to innovate himself out of a job by making the process faster for unmanned probes and safer for future astronauts.
“We can measure the range down to a meter 140 million kilometers away,” Ely says. “For most robotic missions, that’s good enough, but we’ll want a more real-time solution available to astronauts going to Mars.”
Enter the Deep Space Atomic Clock, for which Ely is the principal investigator. If all goes as planned, this uber-precise prototype clock will demonstrate a positioning accuracy as good as that of NASA’s ground-based clocks but needing only a one-way radio signal sent from Earth — or elsewhere — to do it. With a clock so precise that it needs only the one-way transit time, a spacecraft’s computers and/or pilots could manage the rest.
About the size of a four-slice toaster, the clock is one of several instruments on the Orbital Test Bed satellite built by General Atomics Electromagnetic Systems of California and scheduled for launch on SpaceX’s next Falcon Heavy flight under an Air Force-administered ride-share program. Ely thinks engineers can get an operational version of the clock down to the size of a two-slice toaster for real missions.
He thinks the one-way method could even justify a space-based extension of the Deep Space Network, sending out signals from a satellite orbiting Mars, for example.
Ely’s ideal navigation system for Mars-bound astronauts would pair the onboard clock with onboard navigation cameras, the sky-referencing cameras already guiding autonomous navigation in some spacecraft.
The astronauts will “want a navigation solution that relies on multiple data sources,” Ely says. “If you have a failure in any one system, you can detect that.”
The deep space clock works similarly to those at the DSN sites, but is engineered to endure space travel and deliver more precision, says Robert Tjoelker, a NASA physicist working on the program.
Just as in a conventional wristwatch, the time-keeping process begins with an oscillating quartz crystal. But that’s where the similarities end. Electromagnetic fields trap mercury ions in a titanium vacuum tube. Microwaves emitted by the crystal cause some of the electrons in the mercury ions to transition between quantum energy levels.
If the quartz crystal is vibrating at the correct frequency, this interrogation causes electrons to transition to a more excited level. If the frequency varies, however, fewer electrons make the transition. The clock must correct itself.
Designers chose mercury partly because it has a higher transition frequency than the cesium, hydrogen or rubidium in other atomic clocks. This means the quartz can oscillate faster, providing more gradations in time.
If the Deep Space Atomic Clock performs as planned, it will speed up or slow down only 0.3 billionths of a second a day. That would be a 50-fold improvement over the atomic clocks in today’s GPS satellites, according to NASA.
The new degree of accuracy would permit a one-way radio link, whereas today’s method using less precise clocks needs the additional data from a two-way trip.
“A one-way link navigation architecture would significantly alleviate the tracking burden on ground-based assets such as the NASA” Deep Space Network, researchers wrote in their July 2016 academic paper “Mercury Ion Clock for a NASA Technology Demonstration Mission” in the journal titled IEEE Transactions on Ultrasonics, Ferroelectrics, and Frequency Control.
Big chill
Meanwhile, other scientists are wondering whether ultracold quantum gases can lead to entirely new and better ways of steering spacecraft. That’s where the Cold Atom Lab comes in aboard the International Space Station. Inside it, rubidium and potassium atoms will be cooled to within a near-unimaginable fraction of one Kelvin, which is just a hair above absolute zero. At this temperature, the familiar states of matter (solids, liquids, gases and plasmas) no longer apply. The atoms become what’s called a Bose-Einstein condensate in which the atoms act in unison and are sometimes even described as indistinguishable from each other or forming a single quantum entity.
Researchers think that by imaging this condensate and tracking its movement relative to the ISS, they might point the way to development of incredibly sensitive inertial navigation devices such as accelerometers for motion and gyroscopes for rotation, making them suitable for deep space travel.
An advantage of microgravity is that the condensate holds together for several seconds, instead of just a fraction of a second, as would be the case on the ground. That’s long enough for physicists at JPL to observe and work with the condensate in the station’s Cold Atom Lab.
Here’s how the cooling will work:
Magnetic fields first trap a cloud of a billion atoms. These atoms are so sensitive to certain wavelengths of light that turning on the lab’s six lasers brings them to nearly “a screeching halt,” with a force equivalent to 1,000 Gs, says applied physicist Dana Anderson of the University of Colorado. He is founder of ColdQuanta, the Boulder company that built the cooling technology within the Cold Atom Lab. At this point, the temperature has plummeted in less than a millisecond to within a thousandth of one Kelvin of absolute zero.
Then things get really cold.
The lasers turn off, and magnetism holds the atoms in place. Radio frequencies, working in conjunction with this trap, then slough away the hottest of these atoms until so little energy is left that the 40,000 or so remaining atoms are close to a millionth of one Kelvin of absolute zero. These 40,000 take on the odd quantum properties of the Bose-Einstein condensate. The atoms in this condensate act in unison — not unlike the behavior of photons in a laser beam.
But that might not be as cold as things will get. A team of researchers from the University of
Virginia want to see if they can compress and then expand this condensate to chill it to trillionths of one Kelvin. That would be the all-time record for coldness.
The Virginia team also will try to measure the position of the condensate relative to the space station. Here’s how JPL explains the process on its website: “Any residual gravity or acceleration of the ISS will cause the cold atoms to ‘fall’ relative to the station.” Observing this relative motion by camera or “more sensitive techniques such as atom interferometry” could be used to measure acceleration — the word for the forces acting on a spacecraft — to better predict the trajectory.
In further research, scientists think they might be able to take advantage of the fact that when atoms transform into a Bose-Einstein condensate, their simultaneous motion appears wavelike. The lab’s first upgrade, in fact, will seek to take advantage of this phenomenon with the addition of an atom interferometer instrument that will split up beams of atoms by laser and then recombine the beams.
“Each atom separates and recombines, and it shifts the phase of the atom slightly” along the matter wave, says Jason Williams, principal investigator working on the instrument.
Illustrated visually, the atom would appear in a different spot along the line of the wave than if it had never split. Measuring the difference in phases has potential applications for detecting spacecraft rotation and acceleration.
Making history
Flash forward to our notional crew members decades from now, hurtling across the void, perhaps tending to their food crops without fear that they could unwittingly throw off their ship’s trajectory.
If they are students of history, they might know that their peace of mind can be traced to 2018, when the first Deep Space Atomic Clock went to space and scientists pioneered the physics of quantum inertial sensing with the Cold Atom Lab.
Back to the present, work continues toward this future at ColdQuanta’s factory in Boulder, Colorado. Engineers and technicians design and put together the electromagnetic atom traps and glass vacuum cells that facilitate much of today’s quantum research. The company sells a machine for making Bose-Einstein condensates and fills orders for custom equipment. Anderson, ColdQuanta’s founder, notes that one researcher with work slated for the Cold Atom Lab is physicist Eric Cornell who, with two other scientists, won the 2001 Nobel Prize in Physics for making a Bose-Einstein condensate in their lab six years earlier.
As Anderson puts it: “The science has gone into the technology and come back full circle, enabling the same scientists to do new experiments in space.”
The NASA image at the top of the page is an artist’s concept of a magnetic field, also known as an ion trap, and an atom chip in NASA’s Cold Atom Laboratory aboard the International Space Station.
“We can measure the range down to a meter 140 million kilometers away. For most robotic missions, that’s good enough, but we’ll want a more real-time solution available to astronauts going to Mars.”
Todd Ely, JPL space navigator and aerospace engineer

About Amanda Miller
Amanda is a freelance reporter and editor based near Denver with 20 years of experience at weekly and daily publications.
Related Posts
Stay Up to Date
Submit your email address to receive the latest industry and Aerospace America news.
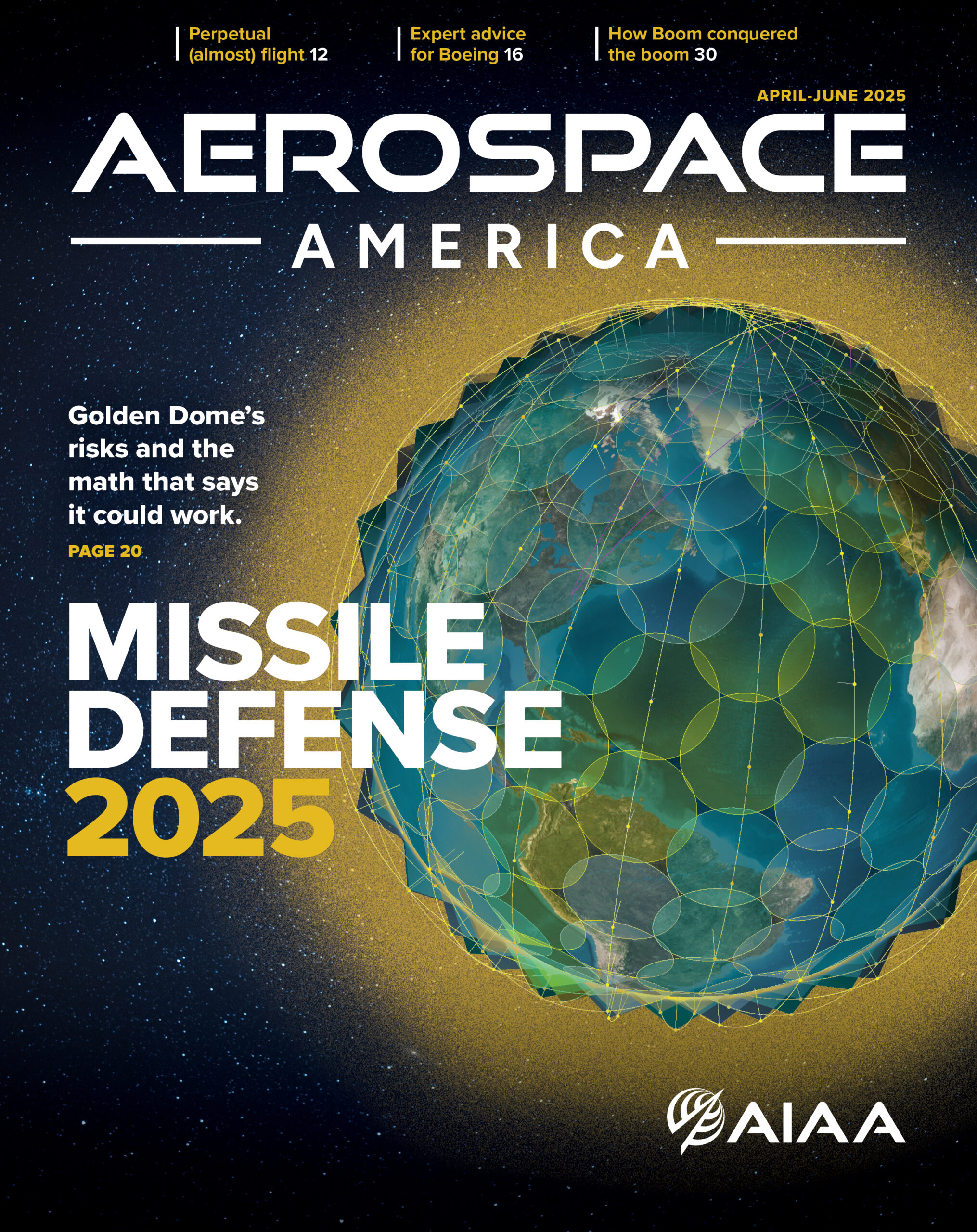
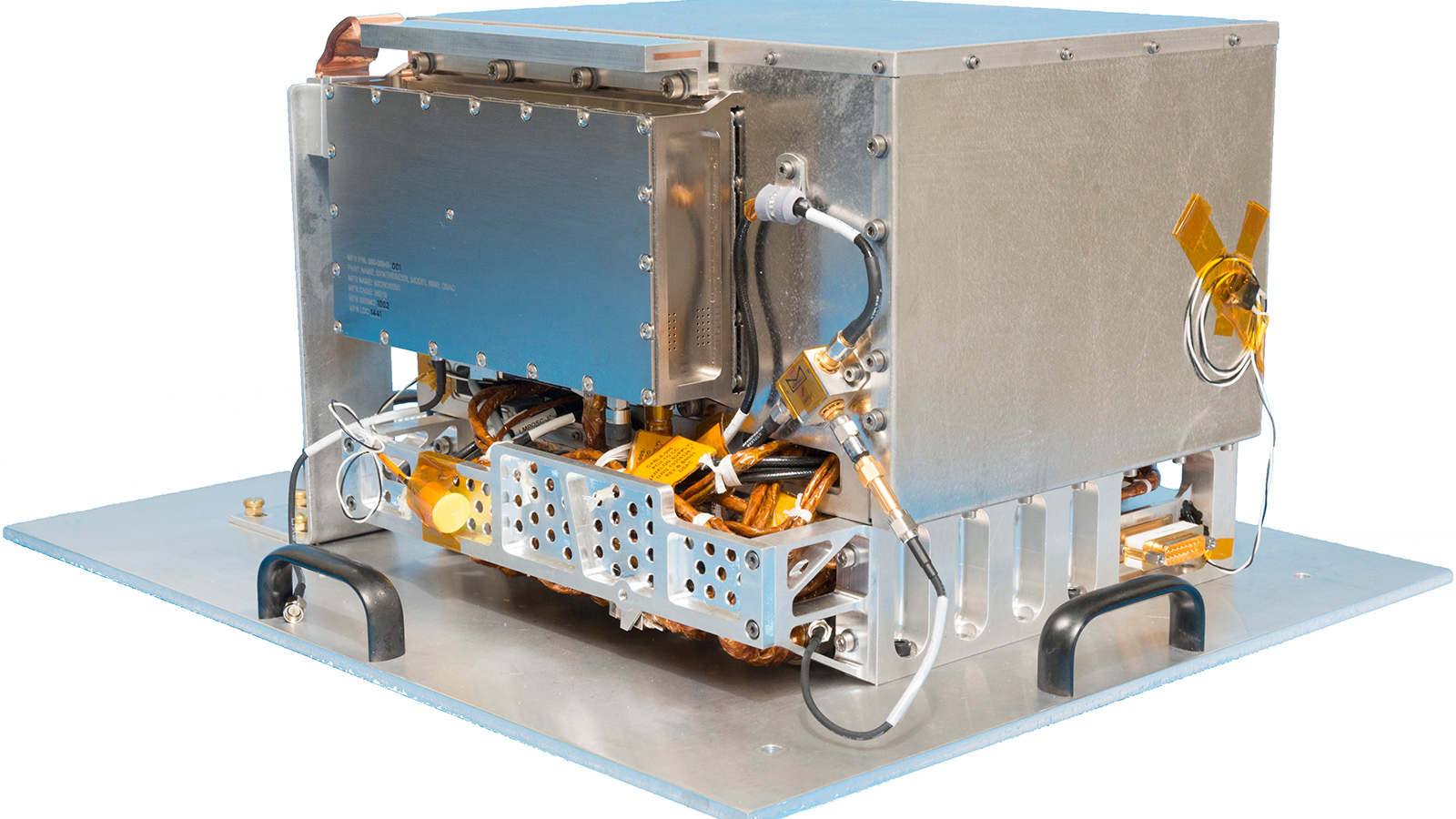
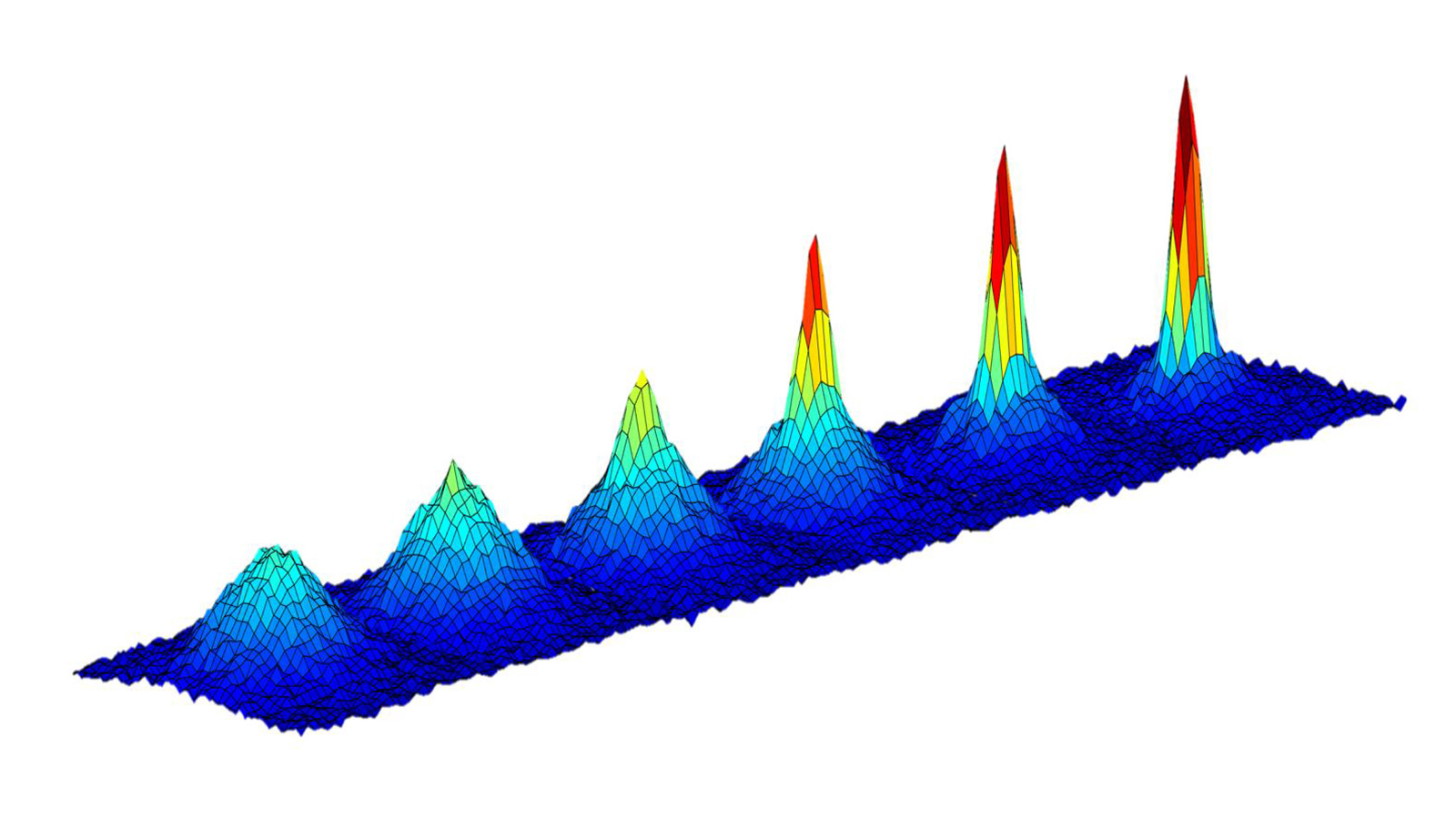
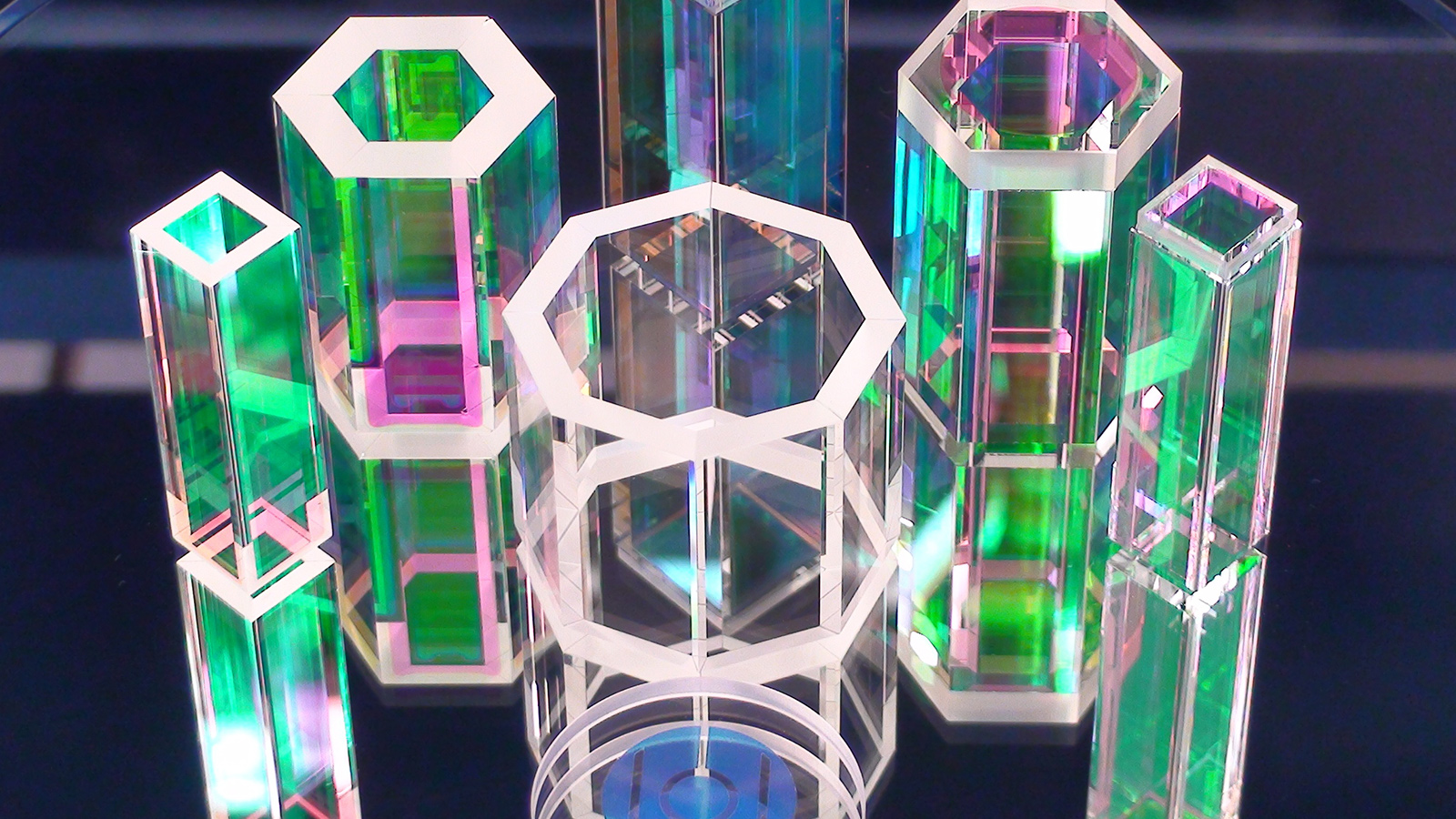