Harvesting sunlight in space
By Paul Marks|April 2023
The emergence of SpaceX’s massive and reusable Starship rocket, alongside advances in robotic spacecraft assembly, could finally fuel the sci-fi dream of spacecraft collecting all the solar energy humanity will ever need and beaming it down to Earth in the form of microwaves for conversion into limitless carbon-free electricity. Paul Marks spoke to the developers hoping to make this dream reality.
★ Click at right to open this story’s Knowledge Guide entries.
★ Click star at right to open Knowledge Guide.
Among those who will be scrutinizing the performance of Elon Musk’s stainless steel leviathan, Starship, if or when it reaches orbit, are two rival but collegial camps of technologists, one based in the United Kingdom and one based in the United States.
Each is determined to liberate us from fossil fuels by launching enormous satellites that, unfettered by Earth’s day-night cycle and adverse weather, would soak up massive quantities of sunlight with solar arrays. The electricity generated would then be converted to microwaves, whose wavelengths would cut through moisture in the atmosphere, to reach giant collector antenna arrays on the ground. There, the microwaves would be instantly converted back into electricity to supply gigawatts of power to our grids.
The rival space solar power concepts diverge greatly from there, but they share two critical, interrelated needs: the ability to manufacture components in vast numbers on Earth and assemble them in space, and an enormous payload uplift capacity to deliver the required supplies and components to the high orbits where they will suck up sunshine.
This is where Starship comes in, with its Super Heavy booster and the Starship spacecraft atop it. With an advertised payload capacity of 150,000 kilograms to low-Earth orbit in reusable mode and 250,000 kilograms in expendable mode, it could be just what space solar power advocates have been waiting for. And Starship’s test flight campaign could be imminent: SpaceX CEO Musk tweeted on March 16 that the first orbital launch attempt would be “near [the] end of [the] third week of April,” provided FAA grants the launch license.
It’s being eagerly awaited. “Starship is going to be a gamechanger in terms of capacity and economics,” says Martin Soltau, co-CEO of Space Solar, a company set up by U.K. government space startup incubator to develop and, eventually, launch a 25-satellite, 50-gigawatt British space-based solar power constellation.
No matter whose concept you look at, a single satellite must be huge to do its job of collecting enough sunlight. On the launch pad, one of the U.K. satellites capable of producing 2 gigawatts of electricity would weigh 2 million kilograms, Soltau says.
If SpaceX can prove Starship’s mettle and enough of the spacecraft are available, Soltau expects that a manageable 60% of the capital expenditure to assemble the constellation would go to launch costs. That estimate, he adds, would not have been feasible in the pre-reusability days, when a traditional expendable space launch cost $20,000 per kilogram to LEO — against today’s $1,500 for Falcon Heavy, and dropping.
“SpaceX is building a large fleet of Starships for their Mars missions,” says Soltau. “Potentially, they’ll have a lot of rockets sitting around with not a lot of revenue generating business, so space solar power could be a huge market for them,”
In fact, he says, Space Solar has already “had some really good conversations with SpaceX,” and the company is “very, very interested in space-based solar power as a potential future launch market. But it’s early days yet.”
SpaceX did not respond to my requests for comment about these talks and its views on space solar power.
Generating astroelectricity
So what exactly do these U.K. and U.S. developers hope to launch? Specifically, a space solar power system would have these essential elements:
- A constellation of highly modular, kilometer-scale solar-collecting satellites, each with a mass of thousands of metric tons, which would be launched piecemeal and robotically assembled in orbit;
- Each satellite harvests solar energy on photovoltaic cells in geostationary, geosynchronous or highly elliptical orbits, where sunshine can be intercepted 99% of the time;
- Electronics on each satellite would convert the solar-generated electricity into a broad microwave beam, which is directed by a pilot beam from the ground, to a fixed point on Earth by the satellite’s phased array antenna;
- For safety, the power density of the beam at ground level must be less than 250 watts per square meter, one quarter the intensity of the sun at its strongest in a cloudless sky at noon at the equator;
- A circa 5-kilometer-diameter antenna array on Earth, which would convert the received alternating electromagnetic microwave energy beam to a direct current, providing gigawatts of electricity.
Such a system could eradicate the debilitating intermittency of today’s terrestrial renewable energy sources: Solar farms don’t work at night or when it’s overcast, and wind turbines can become unexpectedly becalmed, sometimes for weeks, leaving operators returning to fossil fuels. And importantly, by operating way outside Earth’s atmosphere and in almost perpetual sunshine, the receiving stations could reliably deliver what the energy industry calls “baseload” electricity — the basic level of dependable, minimum power an electric grid’s customers demand.
As such, space solar power backers envision a mix of wind turbines, terrestrial solar arrays and their collectors in space eventually combining to make a significant contribution toward greenhouse gas elimination by negating the need for oil, gas and coal-fired power stations.
Such possibilities have not been lost on governments: The U.K. has established the Space Energy Initiative special interest group to pursue space solar power as a means of getting to net-zero carbon emissions by 2050. SEI already has some 75 members — mainly academic, space and energy firms, including the Imperial College London, Airbus Defence and Space, and the U.K.’s National Grid.
“It’s bringing the energy and space sectors together,” says Soltau. “They’re so different, they don’t really talk or understand each other.”
SEI’s program was initiated after Soltau, in his other role as an analyst at British tech consultancy Frazer-Nash, completed a feasibility study for the U.K. government. Soltau and his colleagues concluded that space solar power is “technically feasible, and could be developed by 2040,” given the emergence of reusable heavy launch services and maturity of solar array and antenna technologies, among other factors.
So, plans call for Space Solar Limited by 2030 to launch a 6-megawatt demonstrator satellite capable of beaming power to a ground station, followed by the launch and commissioning of the first 2-gigawatt solar power satellite by 2035. In preparation, SEI members and the U.K. government each plan to contribute £6 million ($7.3 million) toward “derisking” projects designed to get the wireless power beaming and photovoltaic technologies right for the demonstrator. Beyond that, the U.K. government’s Department of Energy Security and Net Zero is also seeking major space solar power investment internationally, and is currently in talks with Saudi Arabia about investing in the project.
Across the pond, the U.S. has a number of ongoing space solar power projects. Caltech in California, for instance, and the U.S. Naval Research Laboratory in Washington, D.C., have already flown space solar power test components in LEO [see sidebar]. The U.S. Air Force Research Laboratory in Ohio is also planning power beaming experiments in space. China and Japan, meanwhile, have had similar programs running for decades and in Australia, a company called Solar Space Technologies is investigating space solar power possibilities there.
And in Europe, at least two tentative space solar power projects are underway: The European Space Agency last year established the Solaris initiative “to prepare Europe for future decision making on Space-Based Solar Power” by 2025. Californian John Mankins, one of the standout space solar power pioneers, says that the European Union’s Horizon R&D program is also considering research on the topic and asked him to give a briefing on its potential. He says the EU was particularly interested in the possibility of beaming space solar power to spacecraft in Earth orbit, cislunar space and to the surface of the moon.
Soltau predicts that although the EU will likely eventually undertake a full-scale space solar program, the U.K. needs its own program. The reason? Post-Brexit Britain does not want to risk being left out in the cold again, as it was over access to the EU’s Galileo navigation satellite network after U.K. citizens voted to leave the bloc. So the Brits think self-determination re: having their own space solar program is the best policy.
Sci-fi origins
Isaac Asimov was the first to posit space solar power in his 1941 short story, “Reason.” The tale followed a rogue robot on Solar Station #5, a “one mile across” solar- energy-collecting spacecraft that sent energy in a tightly focused, searingly hot energy beam to an antenna on Earth. The robot wrests control of the beam director from the station’s human operators, who fear that whole cities could be fried if the droid’s aim is not true.
Asimov’s fiction inspired Peter Glaser of the Arthur D. Little engineering consultancy in Massachusetts to write a paper in 1968 for the journal Science. He found that space solar power was indeed feasible in principle, but not with the technologies of what was then the late-Apollo era. Why? A single satellite, with a 6-kilometer-wide solar collector feeding power via resistance-free superconductors to a 2-kilometer-wide, heavy metal microwave transmitter dish, would have the ludicrous mass of 81.5 million kilograms. Imagine for a moment that someone were determined to launch the necessary components aboard space shuttle orbiters. The cost of doing so in 1981 would have been $85,000 per kilogram, which works out to almost $7 trillion for a single satellite.
In the 1970s and 1980s, the U.S. Department of Energy and NASA revisited the matter but quickly abandoned it due to continuing technology immaturity. But in the 1990s, the idea was examined on paper once again by NASA, which commissioned Mankins, one of its senior advanced concepts engineers, to take a fresh look at space solar power’s feasibility. In a 1997 paper, he concluded that the idea was finally emerging as a serious candidate for power generation.
By 2011, after Mankins had left NASA and established his own consulting business, he was once again commissioned by the NASA Institute of Advanced Concepts, this time to establish the technical and economic viability of a format he’d come up with for a space solar power satellite. Called SPS Alpha, short for Solar Power Satellite via an Arbitrarily Large Phased Array, the highly modular design has become a legendary one in space solar power circles, and Mankins regularly updates it as technologies change. As well as advising on U.S. projects, Mankins has also been consulted by ESA, the EU, the U.K. and the Australian project with Space Solar Technologies, of which he is now a partner.
“The central idea of SPS Alpha was, and this predates by 10 years the megaconstellations, is if you can make really big structures out of modular pieces that can be mass produced, the cost of the really big system can be far lower than you think,” says Mankins.
Those modular pieces would include the solar panels, the microwave amplifiers, the phased array antenna modules, the reflectors and the motors that angle the reflectors into the sun — even the truss structures that hold it all together. This “hypermodular” approach, as Mankins calls it, would allow a satellite to be built on orbit by autonomous robots.
For SPS Alpha, that would mean bringing two million preassembled modules, each weighing 3 or 4 kilograms, from LEO up to GEO, perhaps by ion-powered space tugs. In GEO, an enormous carbon composite framework could then spring open, creating a lattice-like framework into which sandwiched solar arrays or antenna modules could be inserted.
Separate from falling launch costs, the advent of spacecraft mass production means that making those millions is now feasible, a transformation that “is epitomized by the Starlink, OneWeb and Kuiper Systems megaconstellations,” Mankins says.
In an independent analysis, he calculated that each Starlink costs $1,000 per kilogram to produce. In contrast, he estimates that NASA spent $1 million per kilogram designing and building the James Webb Space Telescope “because of the lengths to which the systems engineering and fabrication process go to assure it won’t fail.”
“The two things that SpaceX has done that are extremely important for space solar power are low-cost hardware and long-lived reusable hardware,” he says. “The trick with Falcon 9 is not just that you use it 20 times, but that the hardware itself is mass produced: the engines, the tanks — they’re all cheap. When the Starship flies, whether it’s this month or whenever, it’s the same.”
Soltau raises another advantage: resilience. “Space is a harsh environment where micrometeorites and space weather cause failures. And if you have a hypermodular structure, it’s very, very resilient. A module can fail and it doesn’t affect the modules around it — there are no single points of failure.”
Helix versus heliostats
In addition to Mankins’ SPS Alpha, the second chief space solar power satellite design using modern, resilient, reliable hypermodular construction is the one Britain’s Space Energy Initiative is pursuing. Called CASSIOPeiA, short for Constant Aperture Solid-State Integrated Orbital Phased Array, this design is being developed by Ian Cash, chief engineer at International Electric Co., a wireless power and renewable energy firm based near Oxford.
Both are 2-gigawatt designs, but SPS Alpha would have a mass of 7.5 million kilograms per satellite, whereas CASSIOPeiA would come in at 2 million kilograms. The main reason for this distinction is their different ways of reflecting light onto the solar cells and different ways of orienting themselves in orbit. What’s critical with a solar power satellite is that the solar reflector that directs sunlight onto the solar collector array always faces the sun, while the phased array antenna always faces its designated rectifying antenna — or rectenna — on Earth.
SPS Alpha’s two key elements would be attached to each end of a 5-kilometer-long truss. At the Earth-facing end, a 1.7-km-diameter layer of phased array antennas would face the ground to send the microwave beam Earthward, while a solar panel array sandwiched immediately on top of the antennas soak up sunlight directed to them by the huge device at the other end of the truss: a 3-km-wide cone-shaped envelope containing thousands of steerable reflectors, known as heliostats.
A motor independently adjusts the position of each heliostat to ensure the sunshine is always incident on the solar array, and the cone-shaped envelope also ensures that the solar flux is two to three times higher than pointing the solar array at the sun unaided. Also, having thousands of heliostats provides resilience against space debris and micrometeoroid damage.
Mankins is now up to his third version of a possible SPS Alpha design, which he presented at the 2021 International Astronautical Congress in Dubai. This is the one that he hopes Australia will choose. But something about the design worried Cash, an aerospace engineer and a member of the British Interplanetary Society.
“You’ve still got a multitude of rotating parts there to twist the sunlight, and that means you’ve still got the lifetime reliability issues of having joints and motors and bearings,” Cash says — and all those steerable reflector components make it heavy, too.
So he created CASSIOPeiA as a “solid-state” design with no moving parts that can wear out or cause reliability problems on orbit. Instead, the whole structure would turn slowly as it orbits Earth, with reflectors top and bottom directing sunlight onto a combined solar array and phased-array antenna, which, thanks to the array’s 3D shape, would be able to beam out its microwave signal in any direction to Earth.
The shape that allows this? A helix.
Instead of the flat, circular solar array and antenna structure on SPS Alpha, the 1.7-kilometer helical array Cash proposes comprises 50,000 solar panel and antenna layers. Each layer has a solar panel on the top and bottom, with microwave generation circuitry sandwiched in the middle. The 50,000 layers — they are basically circuit boards, says Cash — in the helical array would be separated by metal rods that act as the phased array antenna elements.
The upshot of this design is that as the constantly illuminated top and bottom solar cells generate power, the phasing of signals to the antenna array steers the beam, in any direction, to the ground antenna. The entire spacecraft, which also lends itself to hypermodular robotic assembly, is rigid, and it only needs to rotate one degree per day as it orbits Earth to keep its reflectors facing the sun, Cash says.
“I think it’s clever, and I understand what Ian is trying to do,” says Mankins of CASSIOPeiA, but he adds that “it’s super, super hard to do.”
Time, as they say, will tell. One independent observer hoping for the success of the space solar power arena is Peter Swan, president of the California-based International Space Elevator Consortium. He also hopes, one day, to supply super heavy-lift for the satellite builders, but without Starship’s emissions. Of course, this depends on a viable elevator tether technology emerging — graphene superlaminate being the latest wunderkind material (after carbon nanotubes failed to live up to their promise).
Swan likes that the U.K. sees space solar power as a great way to generate high-tech jobs, and that the EU, in the wake of Russia’s invasion of Ukraine, seems to recognize that it must ultimately develop sources of energy that it controls.
“You know,” says Swan, the U.K., ESA and the EU “have gotten to the point where they’re asking the question, ‘How do we do it?’ and ‘Should we do it?’ And the answer, of course, is going to come back: ‘Hell yes, we’ve got to do it.’”
Testing space solar power tech on orbit
Two divergent concepts for harvesting sunlight and converting it into microwaves have reached low-Earth orbit in recent years in anticipation of someday beaming microwaves to the ground for conversion to electricity.
The U.S. Naval Research Laboratory’s pizza box-sized Photovoltaic Radio Frequency Antenna Module, PRAM, flew for 30 months aboard an X-37B spaceplane whose mission ended in November. The 6-centimeter-thick tile had a layer of solar cells atop a layer of low-cost radio frequency electronics to convert direct current power to microwave signals. However, no microwaves were broadcast to Earth for fear of interfering with the X-37’s systems or other experiments it was carrying, so the electronics fed radio energy into an onboard dummy antenna, where it was measured.
A big question was how these components would hold up to repeatedly crossing from the hot sunlight back into the cold of Earth’s shadow. “They showed no meaningful degradation over time, even with the temperature cycling of being operated for a good portion of the X-37B’s two-and-half-year mission,” says Paul Jaffe, the principal investigator for PRAM at the Naval Research Laboratory in Washington, D.C. NRL plans to report the PRAM results in October during the International Astronautical Congress in Azerbaijan.
Tiles like PRAM that sandwich solar cells, driver electronics and antennas in one easily replicated unit could one day be installed onto hypermodular space solar power satellites like SPS Alpha, but that is not NRL’s aim. Instead, it is investigating the feasibility of smaller space solar systems beaming power to forward operating bases and disaster zones.
The second concept, Caltech’s Space Solar Power Demonstrator, reached orbit in January via a SpaceX rideshare launch. SSPD’s design departs from the “traditional architecture” of high-mass, high-cost space solar power concepts, says Sergio Pellegrino, the project’s co-director. Caltech envisions ultralight, free-flying, flat structures that could be flown in great numbers, instead of a single, massive, orbiting power station. These structures would consist of 60-by-60-meter ultrathin, flexible composite membranes that deploy on orbit to reveal solar cells on one side, microwave power electronics inside and an underside peppered with microwave transmitting antennas.
Caltech’s SSPD unit is scheduled to begin on-orbit tests later this year once the Momentus spacecraft bus carrying it is fully commissioned, though Pellegrino says their controllers “are talking to their payloads” already. The unit will test 32 types of solar cell for their suitability for use on a membrane, while a flexible microwave transmitter array will attempt free-space, focused transmission to two onboard receiver targets.
SSPD will also test deployment of a 2-by-2-meter flexible membrane, for which Caltech turned to a variant of origami for inspiration, says Pellegrino. “Origami is for folding continuous sheets, whereas kirigami allows cuts within a surface. And we require cuts as we need continuity in certain parts of the surface, but we need other parts to be discontinuous.”
The Caltech team hopes that stored strain energy in the carbon fiber and plastic kirigami-cut membrane will be enough for it to self-deploy. — Paul Marks
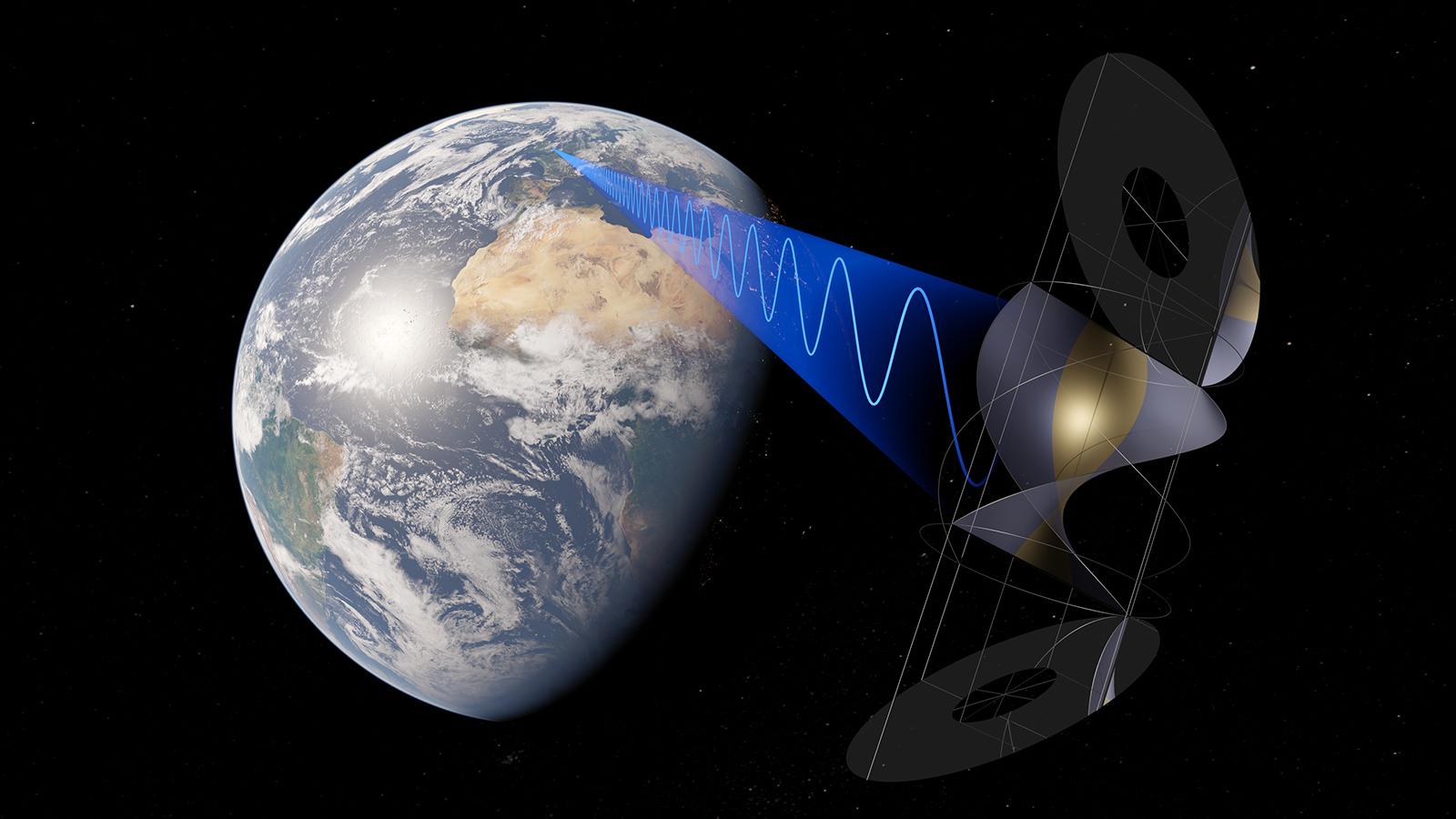
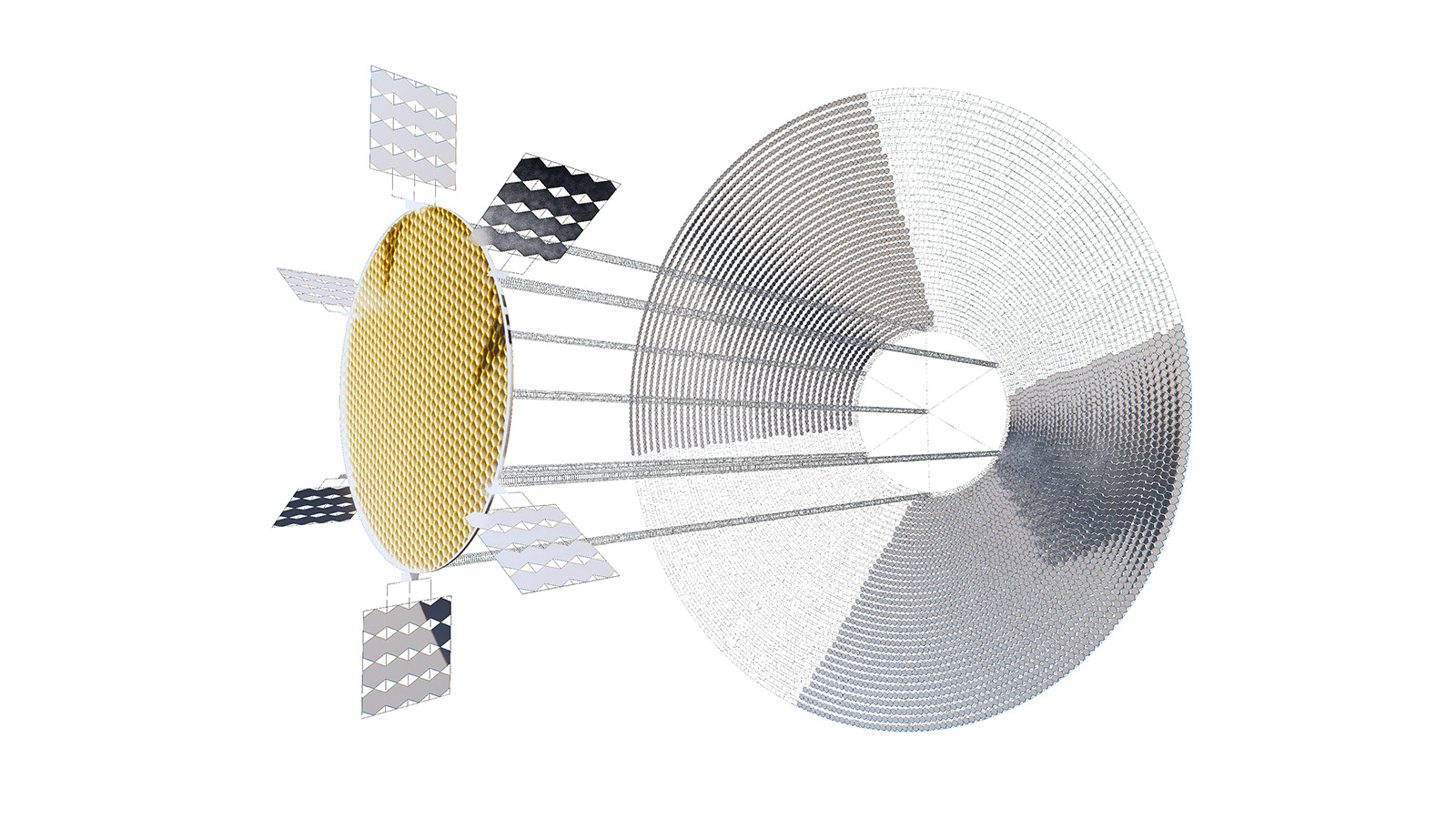
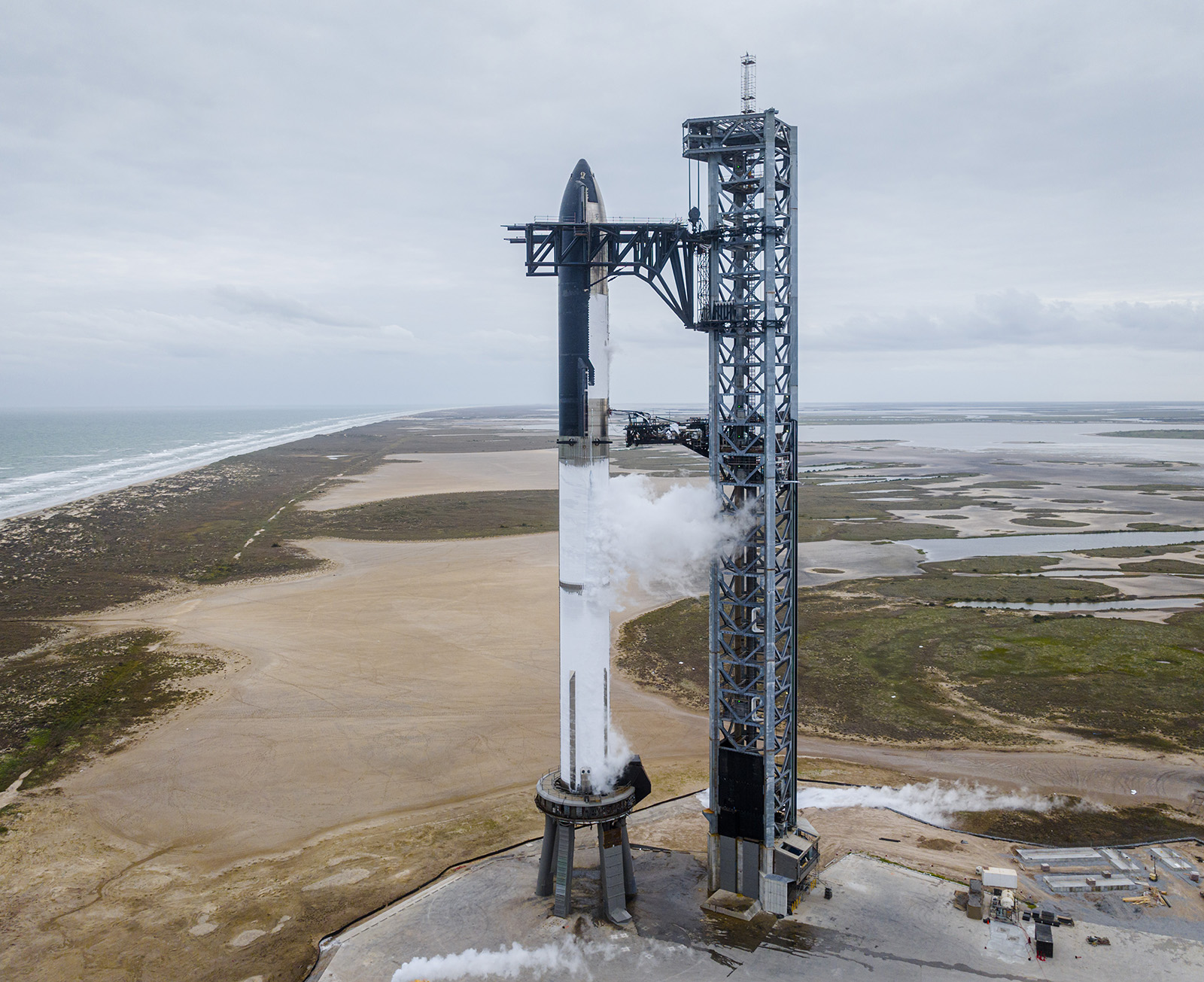