Stay Up to Date
Submit your email address to receive the latest industry and Aerospace America news.
The Transiting Exoplanet Survey Satellite aims to find Earth-like worlds
A little more than a decade ago, astrophysicist George Ricker and his colleagues at MIT had a brainstorm. At the time, he was principal investigator for an international satellite that was operating in orbit as planned but whose core mission of monitoring gamma ray bursts had been outpaced by newer technology. He pitched NASA about possibly repurposing the High Energy Transient Explorer and its instruments to look for transiting exoplanets.
NASA said no thanks, but Ricker came away with a sense that “NASA was interested in doing something more ambitious” on the exoplanet front.
So was Ricker. He began conceptual work toward creation of what we now know as TESS, the Transiting Exoplanet Survey Satellite, a $200 million NASA-funded planet-hunting mission that’s scheduled to kick off possibly in April with the satellite’s launch from Cape Canaveral, Florida.
TESS will record the brightness of individual stars at regular intervals and take periodic snapshots containing hundreds of thousands of stars. These images and data will be downloaded to mission teams at NASA’s Ames Research Center in California and MIT who will flag “targets of interest” through what’s called transit photometry. When the brightness of a star dips for a period of time, that could mean that a planet is transiting in front of it. These targets and the raw data will be released publicly for other planet hunters who will try to learn more about these planetary candidates or identify altogether new ones.
Planetary scientists are counting on TESS’ unique orbit, its focus on relatively nearby stars and its wide-field cameras to speed up the entire planet-hunting business. If TESS can do its job of rapidly assembling a vast numerical trove of likely planetary coordinates, other instruments could then zoom in on the most intriguing candidates and perhaps give humanity its first rudimentary look at an Earth-like world — not decades from now but in just a few years.
The news could come in the form of spectra containing chemical signatures of a planet’s atmosphere. “When someone gets a transit spectrogram, that’ll be a ‘holy crap’ moment,” says astrophysicist Stephen Rinehart, NASA’s TESS project scientist at Goddard Space Flight Center in Maryland.
How quickly could all this unfold? TESS’ designers expect the spacecraft’s first exoplanet discoveries to be validated by mid-2019 on the way to gathering indirect evidence for the existence of 20,000 candidates over the course of the two-year baseline mission. That figure would be a big bump from the current count of some 3,700 confirmed worlds orbiting suns other than our own, which is significant because planet hunting is largely a matter of odds. With that many candidates, NASA estimates that 50 or so might be rocky planets, including a handful in the “Goldilocks” or “habitable” zones around their stars. Those are the terms for orbits that are not so close to the star that any atmosphere or water would be scorched away, but not so far that the planet would be frozen and sterile.
How they did it
If things had played out differently about a decade ago, TESS might not have been a NASA mission. Ricker and MIT at first entered into a partnership with Google, thinking development of the spacecraft might move more quickly that way. Then came the economic recession of 2008-09, which made a corporate funding match seem less secure. Given the interest he sensed from NASA, Ricker reached out to Orbital Sciences Corp., now Orbital ATK, in 2010 to help craft a proposal under NASA’s Explorer initiative, which funds space science missions costing up to $200 million.
Robert Lockwood, now the TESS spacecraft manager at Orbital ATK (headquartered on Warp Drive in Dulles, Virginia) was intrigued by the prospect of finding “an Earth analog.” He volunteered to help put together the proposal.
As Lockwood and Ricker worked on their Explorer pitch to NASA, they had one overarching goal in mind: Set up scientists to deliver the Holy Grail — images or spectra from a possible Earth-like planet — in something less than the decades that conventional wisdom said the feat would take. NASA selected TESS in 2013, just a month before a mechanical failure ended the primary mission of the Kepler space telescope, the spacecraft that took transit photometry to the masses and engendered so much excitement in exoplanets.
A perhaps obvious choice for TESS’ developers was to capitalize on the network of scientists who had sprouted up around Kepler. To stay user friendly, the TESS data will be recorded and presented to the scientific community in the same formats as Kepler’s, and stored in the same repository, now called the Barbara A. Mikulski Archive for Space Telescopes, or MAST, and located at the Space Telescope Science Institute in Maryland.
“Because of Kepler, all kinds of scientists have [software] tools” to detect transits, explains NASA’s project manager for TESS, Jeff Volosin of Goddard. “If you can find it in Kepler, you can find it in TESS.”
Another early decision was to focus in part on what Ricker and other astrophysicists call “naked eye” stars, meaning those that we can see by looking up at the night sky. Exoplanets confirmed around TESS targets would be close enough to Earth for examination by the James Webb Space Telescope, scheduled for launch in 2019. Webb would break down light shining through a planet’s atmosphere to try to figure out its contents. Anticipating Webb’s launch, TESS’ operators will always keep one camera aimed at areas of the sky where Webb will also have continuous viewing.
Before assigning Webb, though, ground telescopes such as those atop Mauna Kea, at Hawaii’s W.M. Keck Observatory, would perform reconnaissance on the target of interest to rule out a relationship other than that of planet and sun, such as a binary star system.
TESS will look most closely for signs of transits across 200,000 bright M-dwarf stars, also known as red dwarfs. These are the coolest, longest-lived and most common in the galaxy. TESS will record their brightness every two minutes and take full-frame images every half hour. The plan is to look at cooler stars because planets orbiting in their habitable zones are presumably closer to the star and circling more frequently. That makes TESS more likely to spot them in its initial two-year mission.
The numbers and longevity of M-dwarfs give planet hunters hope that a well-positioned planet might have developed conditions conducive to life. Scientists continue to model what those conditions might actually be like. One planetary system they study closely is called TRAPPIST-1, located 40 light-years away. Transit photometry suggests that it’s home to seven rocky, Earth-sized planets. Those planets close to the star could harbor water vapor or even liquid water on their surfaces, while those farther away would be icy.
TESS is about “finding the best candidates for characterization and follow-up observation,” says Rinehart, the TESS project scientist.
The TESS team decided early on to look at large numbers of relatively nearby stars through an array of wide-field-of-view cameras. Kepler, by contrast, spent its first years looking at one skinny swath of sky continuously to detect fainter stars about 10 times farther out than those TESS will view.
The TESS team has divided up about 85 percent of the sky into two hemispheres, each containing 13 observational sectors. The spacecraft will spend two orbits, or 27 days, surveying each of these sectors, paying particular attention to bright stars from 30 to 300 light years away.
Achieving that means getting Earth out of the field of view. As the team worked to conceive the mission, low Earth orbit was not an attractive option because Earth would eat up too much of the sky. Following Earth around the sun, like Kepler, wasn’t practical with the Taurus rocket NASA was considering at the time. There was another option, one that Ricker and Lockwood knew about from a technical paper published in 2001, but that to their knowledge no spacecraft had ever used. It’s a kind of elliptical orbit called a 2:1 lunar resonant orbit, resonant referring to the stable time ratio: TESS orbits Earth twice for every orbit of the moon around Earth.
“We worked through this a bit and proved that it would work,” Ricker says.
By following a path that puts TESS in a specific position relative to the moon and its gravity, the orbit should be so stable that TESS won’t need its thrusters to stay on track. In fact, TESS could remain in the same orbit “for decades or longer” without propulsion, NASA says on its TESS website.
Here’s how the lunar resonant orbit will be established: Over the span of 60 days after launch, a series of thruster burns and phasing orbits will put TESS in position to swing around the moon and ultimately onto its elliptical path. The final orbit’s farthest point from Earth will be 373,000 kilometers, with its closest approach of 108,000 kilometers once every 13.7 days.
Another challenge was getting TESS’ voluminous data to the ground as quickly as possible. As it approaches Earth on each orbit, TESS will point its antenna toward NASA’s Deep Space Network, or DSN, a network of ground stations around the globe that will await the spacecraft’s four-hour data downlink. This happens twice in 16 hours to ensure that all of the data is downloaded before the ops team at Orbital ATK wipes the hard drive clean and TESS sets off on another trip.
TESS also will be the first spacecraft on the Deep Space Network to send information over the 26 GHz “near Earth” Ka-band frequency that NASA is just starting to use. This link will be hundreds of times faster than the older, lower frequency near Earth S-band link. The James Webb Space Telescope will be next to come online with the new link.
Once the DSN receives the information, it gets routed for initial processing to NASA’s Ames Research Center in Mountain View, California, while folks at MIT also get a crack at it.
Camera drama
Light enters TESS through the 10-centimeter apertures of its four cameras. Seven glass lenses in each camera then focus it onto charge-coupled device detectors much like those in Kepler and not totally unlike what’s in consumer digital cameras. TESS’ image-processing computer then isolates the data from certain stars and sets aside periodic full-frame images, getting it all ready for analysis on the ground.
The lenses must focus predictably so that incoming photons from different stars — in a field of view the size of the constellation Orion — don’t get too mixed up to reliably distinguish one star from another.
“Once we did our first testing, we noticed the system wasn’t focused the way, to our thinking, it was supposed to be,” says NASA’s Volosin, the TESS project manager at Goddard.
Anticipating that the glass lenses and their aluminum housings would expand and contract at different rates when first getting TESS into space, the instrument designers had glued a rubbery silicone called RTV (room-temperature vulcanizing) between the lenses and housings as a buffer.
“Nobody had used this particular RTV at low temperature, and every rubbery-type product has a glass transition temperature” at which it hardens, Volosin says.
When it came time to test the flight cameras, built by MIT’s Lincoln Lab, in the school’s thermal vacuum chamber, it turned out the RTV hardened sooner — at a warmer temperature — than its manufacturer had indicated, and it tugged on the lenses. “We’re talking microns,” Volosin says. The effect took a week to kick in, one reason the problem wasn’t caught in the prototype phase.
NASA and the rest of the TESS team have decided just to roll with the inevitable change in TESS’ focus that’s going to be triggered — once, and only once, they say — when the cameras go below minus 70 Celsius on their way to their final operating temperature. This creates a shift in which areas of the frame are more sharply in focus while others are less focused.
By design, the lenses were never going to bring in totally crisp images, though. “Since we’re counting photons, it’s built to be an extremely large field of view. We wouldn’t have done that if we wanted to make a crisp picture,” Volosin explains.
“We decided, ‘Well, OK, if [the shift is] going to happen, and it’s a one-time thing, let’s just get it to happen as quickly as we can,’” Volosin says.
The new plan is to take TESS down to minus 80 right away and leave it there. In photometry, cooler temperatures equate to less noise or distortion in the image.
After a year of follow-up tests, a panel of outside experts in January deemed the cameras good to go for launch. The first batch of data should become publicly available around midyear.
Fundamentally, “Kepler was a statistical mission,” says Rinehart, the TESS scientist at Goddard. He doesn’t mean it as a criticism. By looking far away at a single slice of sky, scientists made the astounding calculation that our galaxy likely contains more than 100 billion planets in habitable zones. That realization, in fact, has fueled interest in TESS.
For planet hunters, TESS’ legacy could be something approximating instant gratification.
KEPLER’S RECORD
NASA’s Kepler space telescope made transit photometry mainstream. After its launch in 2009, it pointed continuously at one section of sky for four years on a mission to figure out the prevalence of terrestrial, or rocky, planets in orbital zones potentially conducive to life. NASA credits the spacecraft with finding 2,341 confirmed exoplanets during those years, 30 of those less than twice Earth’s size in habitable zones. Kepler’s main mission ended abruptly in 2013 when the second of its four reaction wheels failed and the remaining two could not keep it pointed correctly. Scientists ultimately figured out how to stabilize Kepler by balancing it against the pressure of sunlight. Getting this technique to work limits the telescope’s field of view to its own orbital plane as it trails Earth around the sun. The mission now known as K2 is credited with confirming the existence of planets orbiting dead white dwarf stars and for finding the first transiting exoplanet in a dense star cluster.
“We are at the beginning of a new era in exoplanet science.”
Stephen Rinehart, NASA’s Goddard Space Flight Center

About Amanda Miller
Amanda is a freelance reporter and editor based near Denver with 20 years of experience at weekly and daily publications.
Related Posts
Stay Up to Date
Submit your email address to receive the latest industry and Aerospace America news.
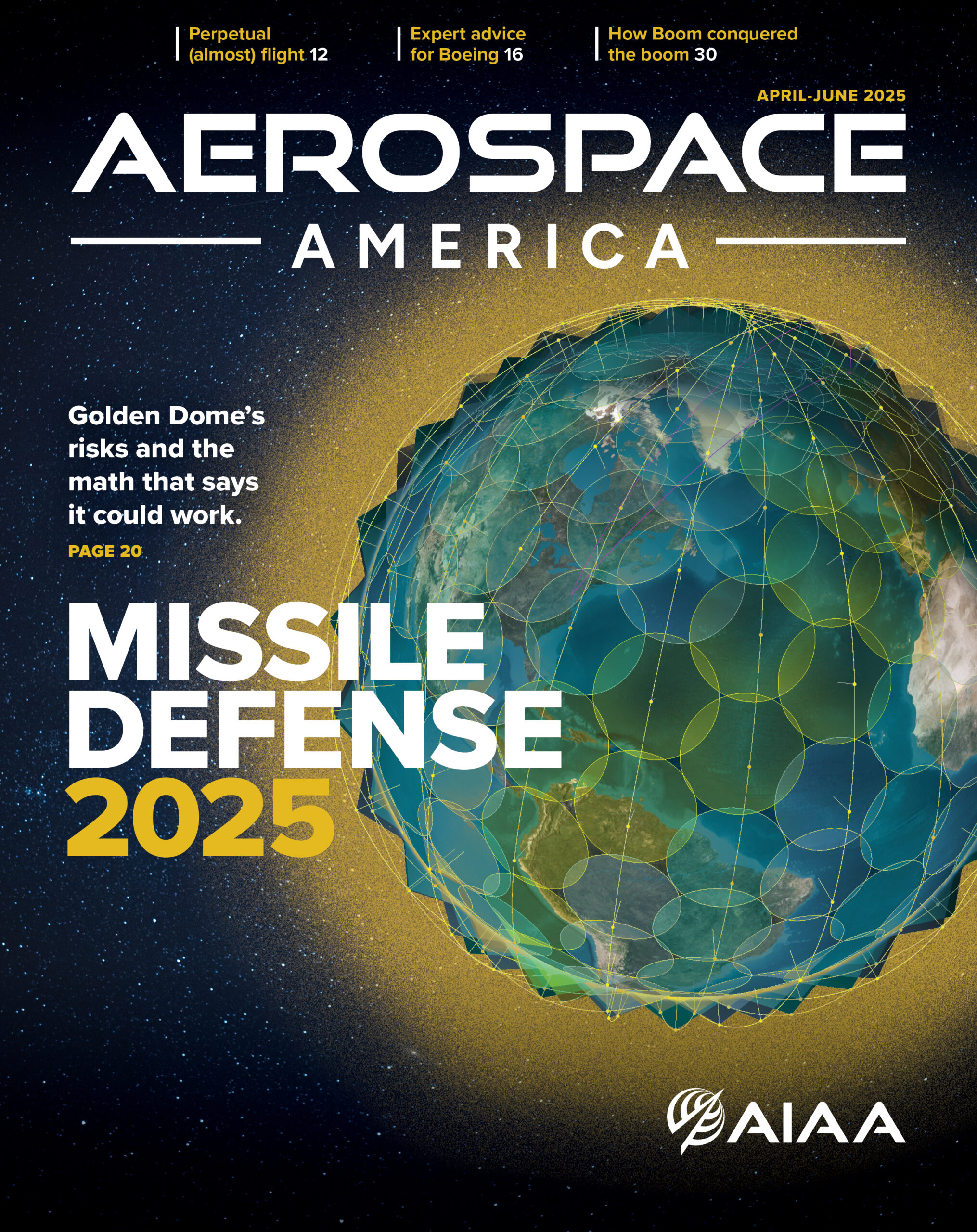
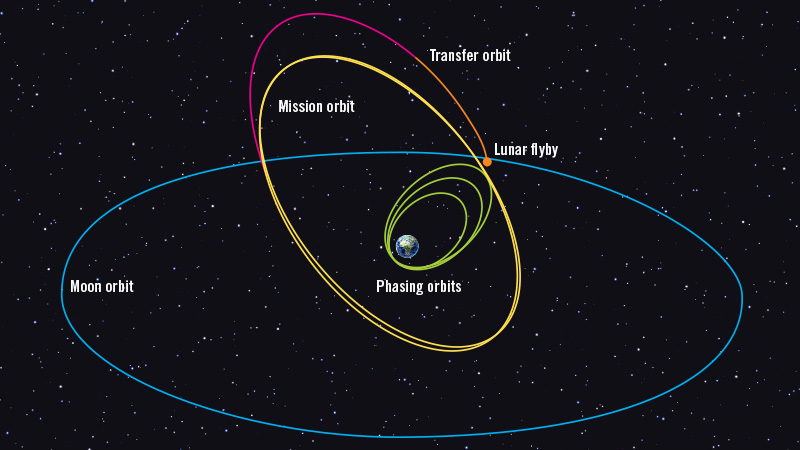
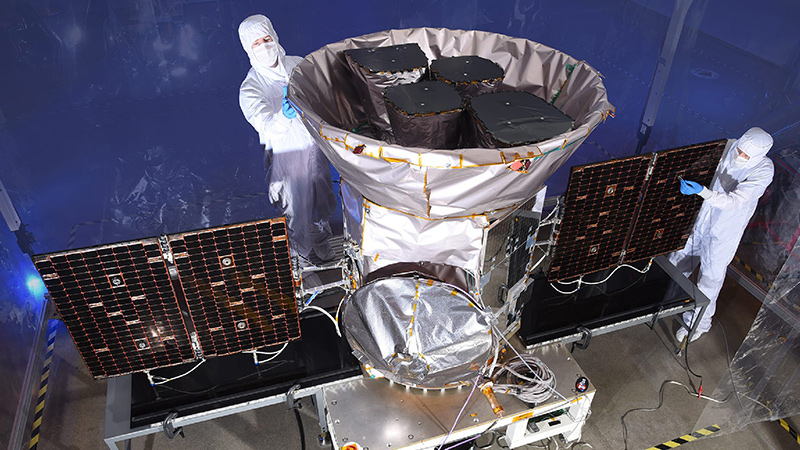
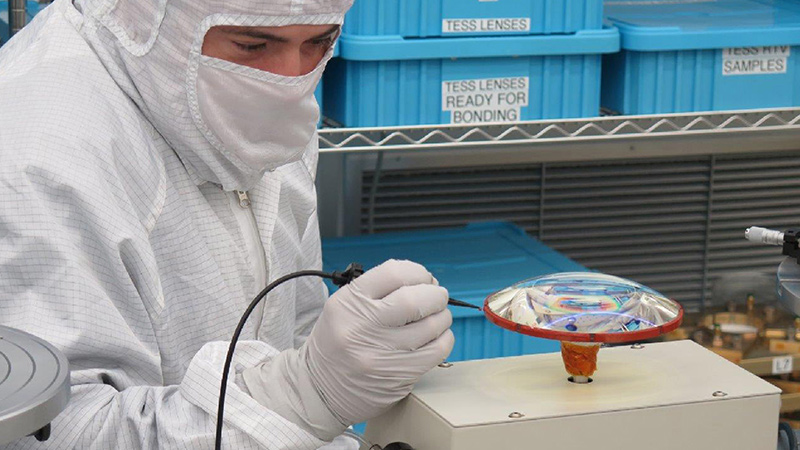