Bringing Mars down to Earth
By Adam Hadhazy|September 2020
The United States and Europe are taking their first steps in a yearslong campaign to deliver rocks and soil from the red planet to terrestrial labs for unparalleled examination. Adam Hadhazy takes us step-by-step through the complex, yet in some ways conservative, mission.
Think of it as an interplanetary “Ocean’s Eleven.” This time, the target is not a casino but a Martian crater holding precious material that could tell scientists whether the red planet once harbored microbes or other lifeforms.
Spiriting away a valuable half-kilogram of this rock and soil will require assembling a team of — yes, 11 — crewless rockets and spacecraft, including the Perseverance rover now on its way to Mars. The team members must all work in concert and in sequence, like the thieves in the 2001 “Ocean’s” film or the 1960 original.
Luckily, NASA and the European Space Agency have more time and budget than Danny Ocean did. The $7 billion Mars Sample Return campaign will unfold over a decade rather than overnight. Success would fulfill a long-held dream of scientists to analyze Martian rocks and dirt in their laboratories. Engineers are improving spaceborne instruments with each successive generation, but even the most sophisticated rover cannot perform the sort of detailed microscopy, imaging and chemical analyses that a scientist can do in a lab, where there are far fewer constraints on size, weight and power.
Pulling off this groundbreaking heist, ironically, will require a highly conservative approach in which technical risk is avoided except where there are no alternatives.
Those in charge of various aspects of the campaign are banking that what has proved its mettle before will do so again. Spacecraft sent to Mars in the past by NASA and ESA were “as complex and capable as the individual pieces of this campaign. So now what we need to do is things we’ve largely done before, but string them together,” says Chris Salvo, the Mars Sample Return systems engineering manager at the NASA-funded Jet Prolusion Laboratory in California.
The overall mission outline is as follows: The first leg began with the July 30 launch of Perseverance (formerly the Mars 2020 rover). After it lands next February, Perseverance will set out to collect and seal samples of rock and soil in tubes and leave them on the surface. Meanwhile, in 2026, a conjoined NASA lander and ESA rover will be launched to arrive at Mars in 2028. This Sample Fetch Rover will roll away, navigate to the samples, pick them up and carry them back to an ascent vehicle positioned atop the lander. Come 2029, the ascent vehicle will boost a container full of the samples into Martian orbit and then jettison it. Next, an ESA-built orbiter — having been launched separately in 2026 — will capture the container via a NASA-provided payload and stow it in a reentry vehicle. The orbiter will fly by Earth in 2031 and release this sturdy vehicle to intentionally crash-land in the Utah desert. Throughout it all, strict planetary protection measures must be followed to ensure that Earthly microbes do not contaminate the sample-gathering equipment on Mars or (albeit far less likely) that Martian life forms contaminate Earth.
It’s a bold mission, no doubt. And it does call for a few “firsts,” such as the first-ever rocket launch from the surface of Mars. “Heritage technology” is the mantra elsewhere.
Like Danny Ocean, managers also must arm themselves with ideas for what to do when events inevitably unfold less than perfectly.
Ain’t broke, don’t fix it
The conservative approach is evident in the first leg, starring Perseverance. Unlike previous new generations of Martian rovers, Perseverance is nearly identical in size and mechanics to its immediate predecessor, Curiosity, which landed on Mars in 2012. An exception would be its wheels, which are made of a stronger aluminum and have a gently curved tread pattern versus Curiosity’s chevrons. These new wheels should grip just as well as Curiosity’s but better withstand the pressure of rocks that led to the holes and tears experienced by Curiosity. Naturally, Perseverance also packs improved instruments, plus a minihelicopter, dubbed Ingenuity. Perseverance’s engineers at JPL made liberal use of spare parts and components left over from Curiosity’s construction, including its descent stage and avionics, as well as sticking with the sky crane touchdown system which, although highly innovative and unproven on its first go-round for Curiosity, was a thorough success.
Perseverance’s destination — and thus the site where return samples will be collected — is Jezero Crater. It’s an appealing destination, with orbital imaging revealing that a river once flowed over the crater’s rim and formed a lake, creating a once-potentially habitable environment for microbes or other simple organisms. As Perseverance roams the region, scientists at JPL and various universities will look through the rover’s mechanical eyes and select compelling sites for sample collection. These sites would, for instance, include those holding minerals, such as clays, that can only form in the presence of water, plus rocks that might preserve chemical markers of life that existed eons ago.
Careful, deliberative gathering of samples will establish geological context for the rocks, meaning where they formed or deposited, and in what conditions, which is key for scientifically evaluating the information the rocks contain. “Geologists want to know, ‘What’s the story here?’” says Salvo. “That’s why geological context is so important.”
That critical context is in contrast to the random bits of the red planet that have already made their way to Earth as meteorites. Such skyfalls will never rival the scientific value of targeted samples brought back to Earth. Meteorites often typically spend millions of years being irradiated in space — and that’s before they go through the fiery crucible of atmospheric entry and ground impact; hardly an ideal journey for preserving delicate traces of life.
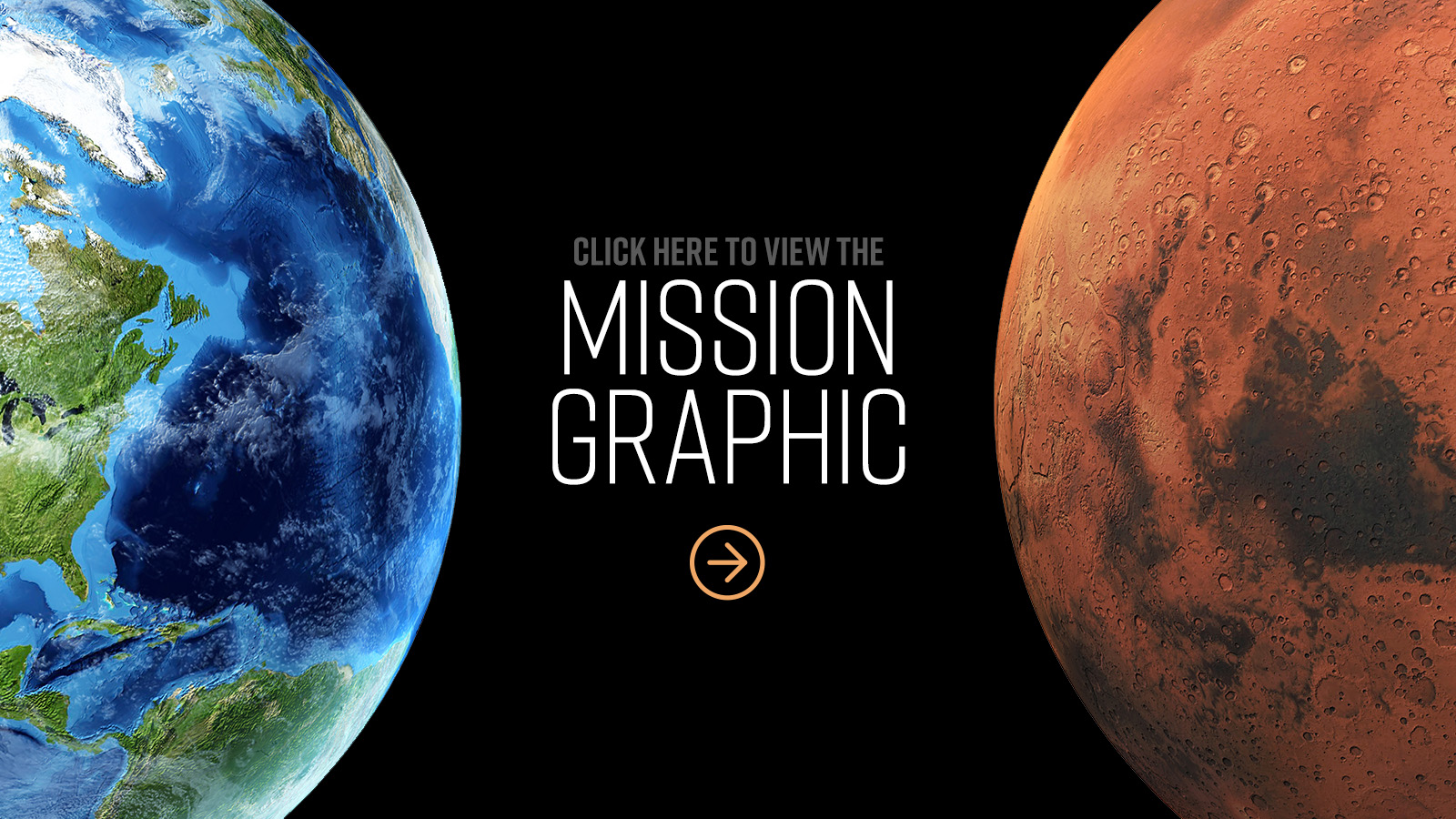
As Perseverance rolls along, the science team will look for rocks or regolith — meaning broken-up rocks and dirt — that look worn by ancient water. If the target is a rock, Perseverance can scrape it to reveal what’s below or drill into it to extract and preserve a 15-gram core sample in a sample tube. This coring and collecting is different from Curiosity, which instead pulverizes rock with its drill. The coring process begins when a mechanical carousel on Perseverance’s belly rotates to put a drill bit and pen-sized titanium sampling tube within reach of T. Rex, the nickname for the short sample handling arm on the rover’s belly. This arm installs the tube and bit into the drill on the end of Perseverance’s main robotic arm, which bores as deeply as 5 centimeters. T. Rex returns the filled tube and bit to the carousel, which rotates to deliver the tube to inspection and sealing stations. The rover will carry a series of such hermetically sealed tubes of samples on a rack until the scientists identify a safe location to deposit them on the surface, where starting in 2026 they will be picked up by the Sample Fetch Rover. Plans as of now call for establishing several of these depots to cache a total of 30 samples of rocks and soil.
Fetch, rover, fetch
The fetch rover, whose first parts should be in production by Airbus before 2021, will draw heavily from the specs for ESA’s ExoMars Rosalind Franklin rover, slated for launch in 2022, but at 230 kilograms, it will be about half Franklin’s mass and not quite a quarter of the mass of Perseverance.
“The fetch rover is just fetching,” says Ludovic Duvet, a senior system and technology engineer at ESA who led the Sample Fetch Rover’s development. “It’s purely a courier.” In fact, it won’t have science instruments, but the black-and-white cameras on board, including a camera at the end of the robotic arm, will gather a substantial amount of ground-surface imagery, which could help show local changes to environments where Perseverance has roamed just a few years prior. The locomotion system’s readings of wheel-ground interactions should also be an interesting source of geological information, says Duvet, when correlated with in-situ and orbital images. As for those wheels, the fetch rover will ride on four, 7-cm-diameter, mesh-like wheels designed by NASA’s Glenn Research Center in Ohio that are adaptable to uneven terrain and lightweight for their large size.
A solar panel will power the rover, giving it about a six-month window to receive precise location information recorded by Perseverance and Mars orbiters for the sample tubes before the Martian winter sets in and starves the rover of light, putting an end to its fetching days. Salvo compares the rover’s quest to a treasure hunt, where an “X” marks the spot of each tube. To enable recovery, the rover will rely on its cameras to autonomously detect tubes by their shape and contours, determining their orientation for subsequent pickup by its robotic arm and gripper that will load them into a storage rack onboard.
Should the Sample Fetch Rover not reach Mars or break down on its way to the samples, Perseverance will also be able to retrieve its own dropped samples. “It’s just one more way to build robustness into the mission,” says JPL’s Salvo.
But if all goes as planned, the fetch rover will return the maximum 30 samples to the lander, which will be a scaled-up version of prior landers. Design cues have been borrowed from NASA’s InSight probe, which arrived on Mars in 2019, and which itself was based largely on the Phoenix lander that alit in Mars’ north polar region in 2008. Further harking back to those landers, the entry, descent, and landing of the sample fetch vehicles will involve the tried-and-true method of a heat-shielded aeroshell plowing through the atmosphere, followed by the deployment of a parachute and jettisoning of the heat shield, culminating in a final thruster burn to soften the touchdown. Because of their smaller size and mass compared to Curiosity and Perseverance, the fetcher and lander won’t have to descend to the surface on a tether via the sky crane technique. “It’s all based directly on what we’ve done before,” says Salvo.
Rendezvous in space
Upon return to the lander base, the fetch rover’s robotic arm will lift a door to the sample container, ensconced as a payload within the Mars Ascent Vehicle atop the lander. The rover will place the tubes in slots inside the container, with the number of slots giving the interior a honeycomb-like appearance. Once loaded, the container will close shut, and the Sample Fetch Rover will back off to a safe distance from the lander to video the subsequent launch. In case of a mission-dooming launch failure, the filming would serve as a means of diagnosing what went wrong. But more optimistically, the filming will instead capture humanity’s historic first rocket launch from another planet. “That will be a nice moment for everyone,” says Duvet.
As currently conceived, says Salvo, the Mars Ascent Vehicle will be a two-stage rocket fueled by an industry-standard, solid propellant that can lay idle for years and withstand the temperatures in the Jezero Crater region. To fit inside the lander, the launcher must be small — no taller than three meters — but given that the gravity on Mars is just 38% of Earth’s, a model-rocket-like device will suffice. In April, NASA announced its intention to procure the launcher’s solid rocket motors from Northrop Grumman.
Once in orbit, the ascent vehicle will spit out the sample container, which will be roughly spherical and about the size of a basketball. Shortly after, a NASA-provided payload on ESA’s Earth Return Orbiter will open a flap-like door and engulf the container in a slow, steady maneuver. “Basically, the canister will swallow the ball, like a giant fish,” says Salvo.
In the event that the orbiter, already in construction by Airbus and Thales Alenia Space, cannot rendezvous with the container, the container can remain in Martian orbit for years to await another attempt.
For all this to go smoothly, the launches and transits must be carefully orchestrated. Plans call for a new European launcher, the Ariane 64, to launch the orbiter shortly after the lander goes up in 2026 on an as-yet-unspecified U.S. rocket. The orbiter would overtake the lander en route to arrive first and serve as a communications relay for the surface elements of the mission. This is a necessary precaution, given that there is no guarantee that today’s Mars orbiters that serve that function will still be alive and kicking as the 2020s wear on.
The orbiter will have a hybrid propulsion system consisting of an electric propulsion system, like that which propelled a prior Airbus spacecraft built for ESA, the BepiColombo probe to Mercury, as well as a conventional chemical engine to do a big burn for orbit insertion upon Mars arrival, plus the usual maneuvering thrusters. The orbiter will also come equipped with a precision guidance, navigation and control system as well as optical sensors, all working in concert to enable visual acquisition and a rendezvous with the orbital sample container. If that sounds rather tricky, Kelly Geelen, the ESA Earth Return Orbiter systems engineering team leader, assures it is in hand, with many technology demonstrations already completed. “We feel comfortable,” Geelen says. “The vast majority of the equipment is at a high technology readiness level.”
Earth for Earthlings, Mars for Martians
Mission planners must guard against any possible backward contamination of Earth from life forms on Mars.
“While we don’t think there’s a strong chance that the samples we get from the top couple inches on Mars are going to have anything viably biologically active in them,” says Salvo, “out of an abundance of caution, the position that NASA, and really the world, has taken is to be prudent.”
Within the lander on Mars, the sample container will incur some degree of contact with Martian air and dust. So even though the container will be subjected to the vacuum and radiation of space after ejection into orbit by the ascent vehicle, the container will still be treated as “dirty,” says Salvo. Accordingly, once it is engulfed by the orbiter’s canister, the surrounding volume will be considered a dirty zone, and the sample container must somehow be transferred into a sterilized zone. NASA is still working out some of the details for how to do this, but the general concept calls for shunting the container into a sealing vessel, with its exterior possibly heat-treated in the process. That vessel then will be shunted into another sealing container as it moves into the Earth Entry Vehicle, the flying-saucer-shaped vehicle that will crash-land in Utah. ESA’s Duvet compares these multiple levels of containment to Russian nesting dolls, with the result being that multiple layers isolate the samples from eventual contact with the external, terrestrial world. “We are taking very careful steps to break the chain of contact with Martian surface materials,” adds Salvo.
Once safely stowed, the samples will be ferried from Mars back to Earth by the return orbiter. The orbiter will conduct an Earth flyby to release the entry vehicle and then perform an abort-style maneuver to get out of the way of Earth, Geelen says, and enter a heliocentric orbit that will avoid any repass of our world for a century, thus providing another level of planetary protection.
The entry vehicle will scream through Earth’s atmosphere, destined for a controlled hard landing on the Utah Test and Training Range. This vast, dry lakebed 130 kilometers west of Salt Lake City is the same landing zone where NASA’s Stardust probe came down with its cometary and stellar dust in 2006 and where the OSIRIS-REx container should arrive in September 2023 with its material from the asteroid Bennu. Both those missions, though, relied and will rely on a parachute to slow the entry vehicle’s descent. This time around, because of planetary protection concerns, not to mention possible damage to the samples, designers decided that the capsule must be ready to withstand a parachute failure that, consequently, eliminated any need for a parachute to begin with, Salvo says. Accordingly, the entry vehicle will intentionally plow into the ground. Like modern cars, the vehicle is being designed with crumple zones — areas of its structure meant to shock-absorbingly compress — that will protect the precious red planet cargo. Then the hands-on science begins. In much the same way that significant science is still being squeezed out of the moon samples snatched by Apollo astronauts half a century ago, the Mars samples would be the scientific gifts that keep on giving as engineers continue to advance Earthly instrumentation and analysis techniques.
“With sample return, you’re not constrained to those instruments you can carry on your back to Mars, and you’re not constrained in that moment [of time],” says Salvo. “That’s why this mission is so compelling.”
Staff reporter Cat Hofacker contributed to this report.
Related Topics
Space Science“While we don’t think there’s a strong chance that the samples we get from the top couple inches on Mars are going to have anything viably biologically active in them, out of an abundance of caution, the position that NASA, and really the world, has taken is to be prudent.”
Chris Salvo, NASA-funded Jet Propulsion Laboratory
Review board to examine campaign
NASA in mid-August announced the creation of an independent review board, or IRB, to examine whether the Mars Sample Return is feasible within the budget and timeframe. The IRB, which will be led by former Orbital ATK President David Thompson, will spend eight weeks in discussion with the European Space Agency and NASA about the mission, then submit a final report and recommendations to NASA. Recommendations from similar review boards have prompted changes to individual missions before construction begins in earnest. A review of the Nancy Grace Roman Space Telescope (formerly the Wide Field Infrared Survey Telescope or WFIRST) completed in 2017 led NASA to reclassify the coronagraph instrument as a technology demonstration in an effort to reduce the amount of testing needed and total cost.
— Cat Hofacker
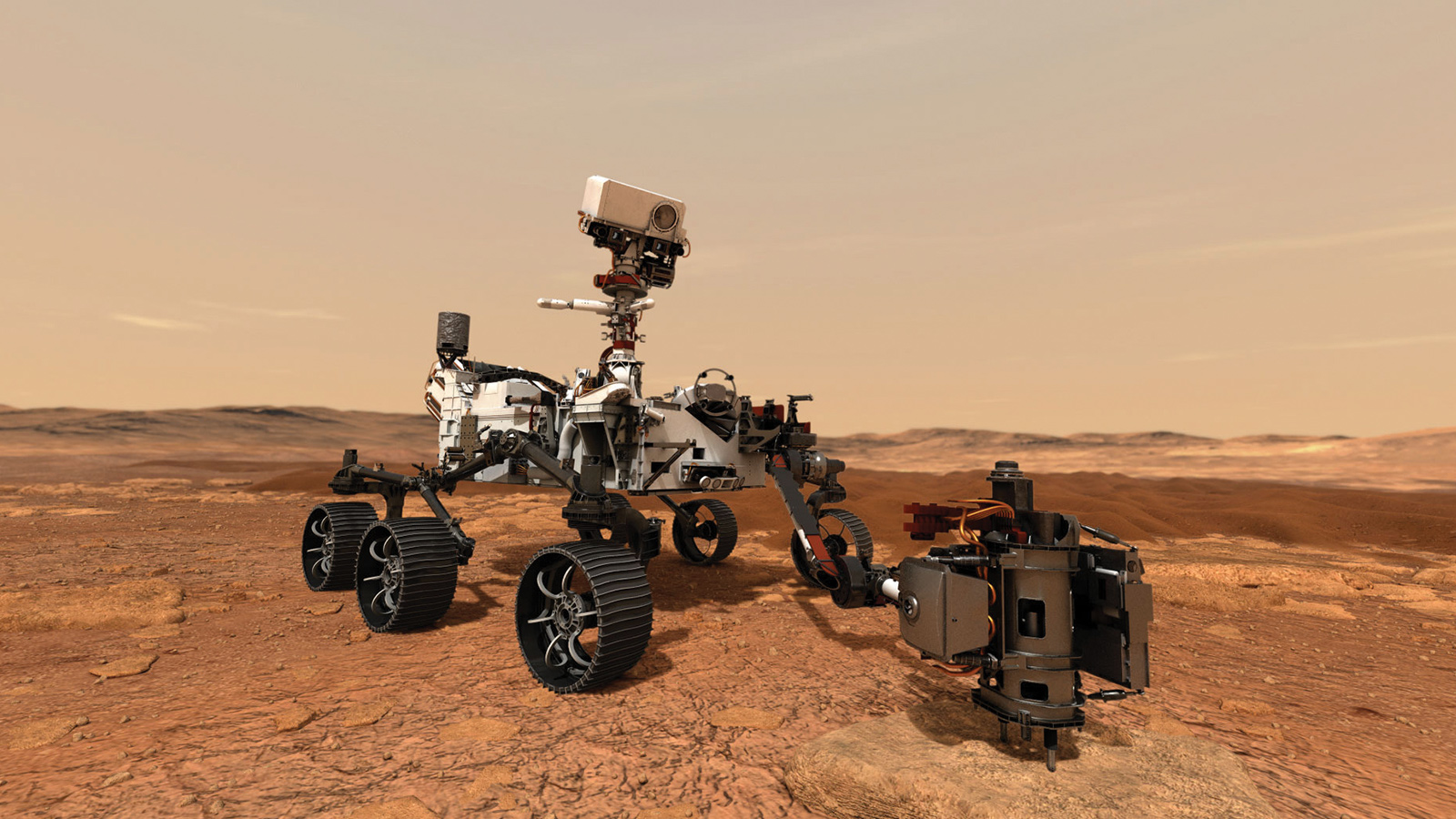
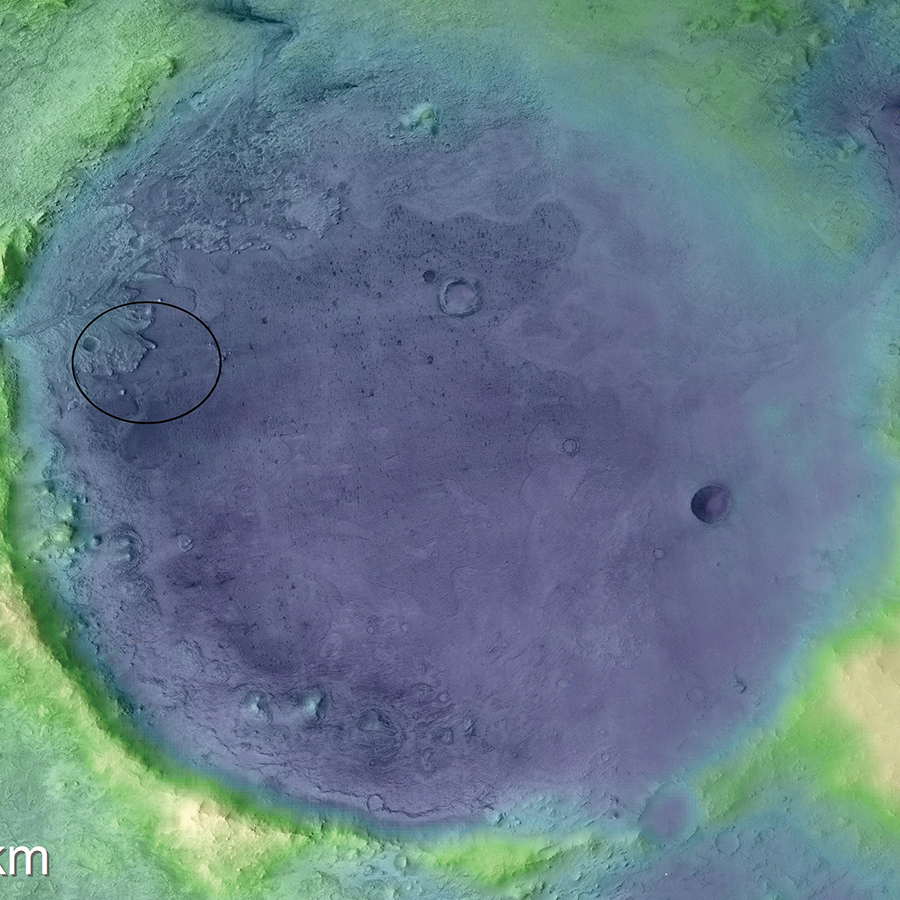
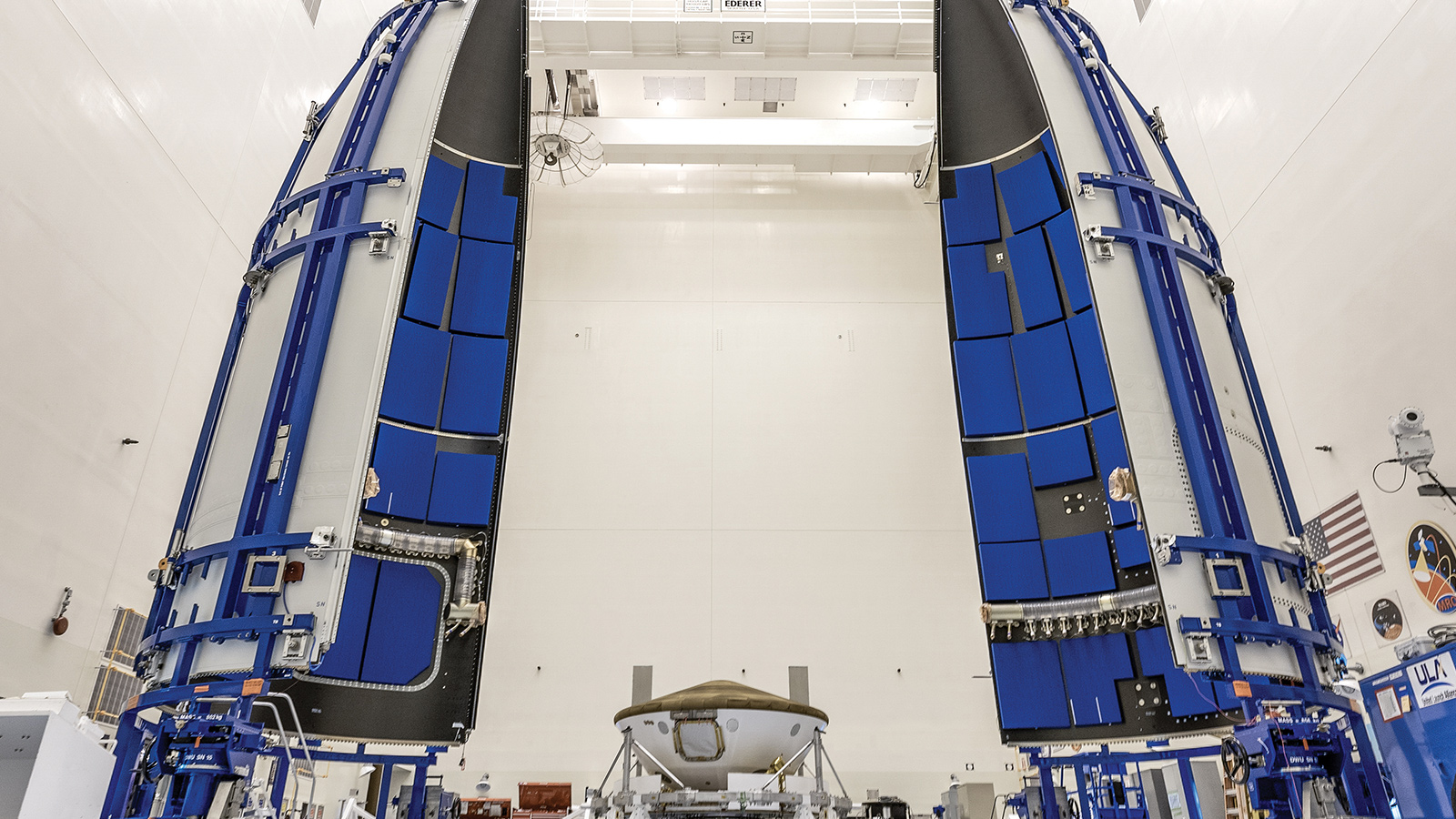
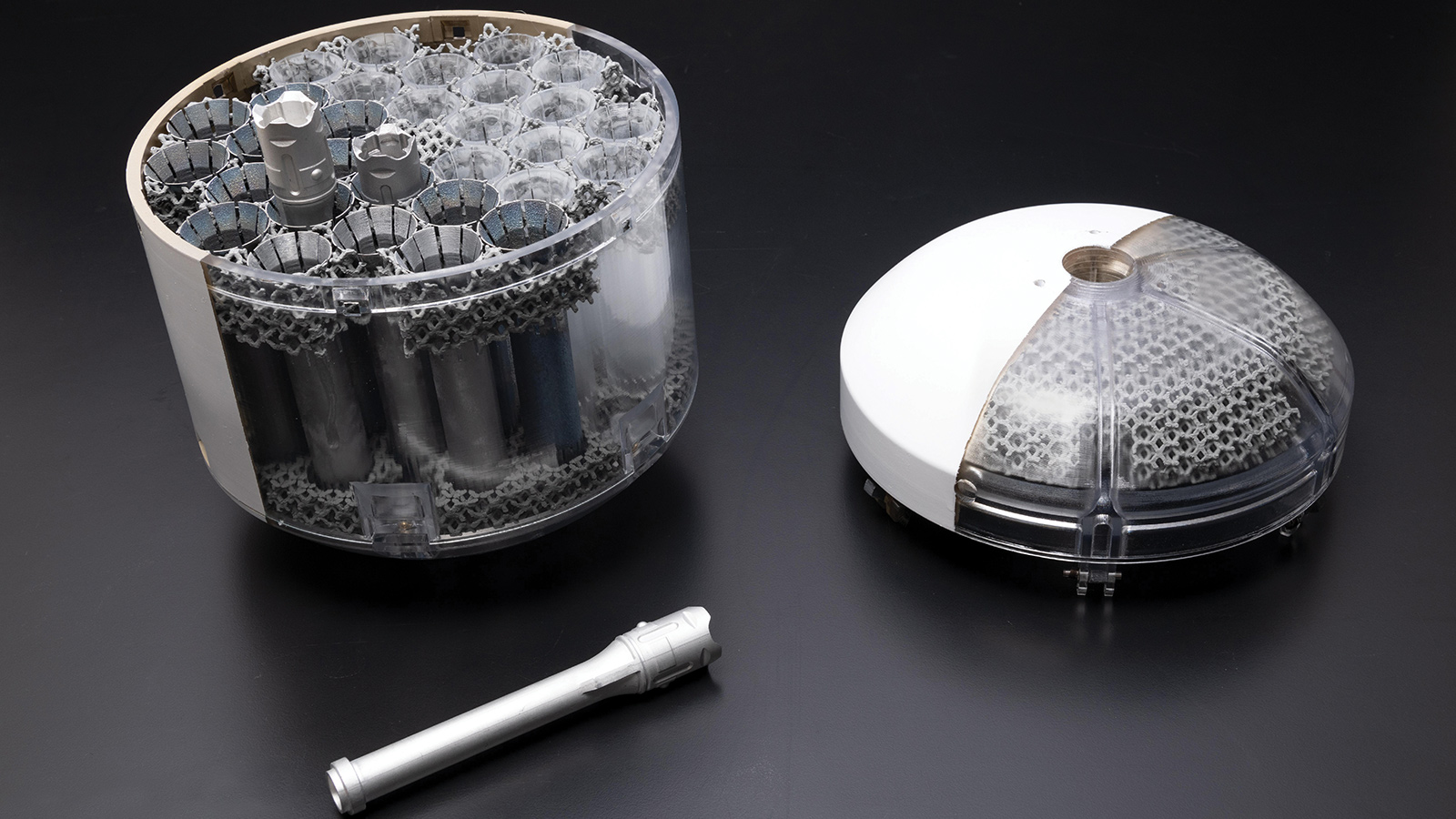