Building a new astronomy tool
By Amanda Miller|April 2021
X-rays emitted by black holes, quasars and other features in deep space have a story to tell, but astronomers have yet to fully tune in by examining the polarization of the beams. Amanda Miller tells the story of a space observatory that could do just that after its launch later this year.
★ Click at right to open this story’s Knowledge Guide entries.
★ Click star at right to open Knowledge Guide.
The Imaging X-ray Polarimetry Explorer will be an odd-looking astronomy spacecraft when it reaches Earth orbit and morphs into its final form. Three cylindrical telescopes perched at the end of a 4-meter-long extendable boom must reflect X-rays from the cosmos to detectors on the main spacecraft to turn photons into images and measurements.
The design is unusual for a space telescope, and so is the story of how NASA, the Italian Space Agency and contractor Ball Aerospace have arrived at the pre-launch testing phase of what will be the first space observatory dedicated exclusively to X-ray polarimetry, the art of measuring the orientation of energy oscillating within incoming light beams.
First, though, to visualize polarimetry, imagine you could see X-rays coming straight toward you. If they were polarized, you’d see the energy oscillating on the same plane in contrast to unpolarized X-rays, in which the energy would be oscillating on multiple planes. The orientation of this plane is the direction of polarization, and it could be vertical, horizontal, or anything in between. IXPE must measure this direction of polarization, and it will do so by absorbing the X-rays in a gas and imaging the tracks of electrons that are expelled when those gas molecules absorb photons. By gathering many of these electron tracks, the scientists will attempt to statistically determine the direction of polarization for a region of the sky and create images of cosmic features based on where the tracks start.
The resulting cosmic maps should provide clues about the kinds of cosmic structures or phenomena that produced the polarization characteristics.
Overall, X-ray polarimetry could add a new tool in astronomy’s long-standing effort to compensate for our inherently one-sided view of the cosmos. Telescopes soak up radiation in a host of wavelengths as it arrives from deep space. “We can reconstruct what we think something looks like in three dimensions even though we don’t see in three dimensions,” says astrophysicist Martin Weisskopf of NASA’s Marshall Space Flight Center in Alabama and IXPE’s principal investigator.
For five decades, Weisskopf has dreamed of adding X-polarimetry to that mix, or simply put, “data that has never been taken before.”
Creating a spacecraft to do that required devising a method for precisely aligning the telescopes with the detectors, creating the most sensitive X-ray detectors yet, and weathering two surprising programmatic twists.
Launch vehicle surprise
Initially, the IXPE team assumed that their spacecraft would need to fit inside a bargain-priced, air-launched Pegasus XL launch vehicle. After all, NASA had selected IXPE in January 2017 as the next in its line of Small Explorer missions whose budgets are capped at $200 million. IXPE’s estimated program cost came in at $188 million, including the launch, the contract with Ball Aerospace, and two years of operating the observatory at the Laboratory for Atmospheric and Space Physics in Colorado.
The Pegasus XL drove the design work throughout Ball’s runup to the July 2019 critical design review in front of NASA. The thinking was that IXPE would need to fit inside the XL’s 2-meter-long, 1-meter-wide fairing, meaning it would be phone-booth-sized when stowed. That was a problem, because the three telescopes had to be held in front of the detectors by a precise distance of 4 meters, the focal length created by the wide, glancing angles of concentric, cylindrical mirrors inside each telescope.
But a spacecraft of that length wouldn’t fit in the XL. So the engineers chose Northrop Grumman’s Coilable Boom technology, which has a history going back decades.
Once IXPE is in orbit, the LASP controllers will command three bolts to release the boom.
Stowed coiled up in a canister, compressed to a length of 290 millimeters — about one-thirteenth of the boom’s final length — the boom’s three rod-shaped fiberglass longerons, forming its backbones, will gradually release their springlike tension to unwind to the full 4 meters over the course of about three-and-a-half minutes. Then over the next minute and a half, the boom will gently oscillate as it settles into position, its flexibility protecting it from snapping or jolting the telescopes too hard.
Shortly after NASA approved this design came a surprise from the agency: IXPE would now be launched on a Falcon 9 rocket with its larger payload fairing. Shifting to a fixed boom, rather than an extendable one, might be possible, but would it be wise?
This twist prompted what Ball Aerospace’s program manager for IXPE, MacKenzie Ferrie, calls an exercise in “engineering economics.”
Switching to a fixed boom was tempting. As Weisskopf put it: “Anyone associated with NASA will tell you that anything to do with moving parts and space is always something to worry about.”
In the end, the team decided that the cost and schedule limits would not have allowed a redesign.
The engineers also needed to ensure that IXPE’s telescopes could direct the X-rays to each telescope’s detector with the required precision.
They devised a threefold plan for precision: Determine how much margin the 15-millimeter-by-15-millimeter detectors had for alignment; test the deployment of the boom on the ground to see if the alignment with the detectors fell within that margin; and, just in case, install a motorized tip-tilt-rotate mechanism joining the boom and the telescopes’ main support structure, or payload deck, so the alignment could be adjusted in space, if necessary. After deploying the boom in Ball’s Boulder factory, Ferrie thinks it can be deployed within the margin and might never need a mechanical adjustment.
Previously, the engineers had thought about tracking the alignment with lasers but calculated that more equipment would add costs and that making multiple adjustments would induce wear and tear.
“You don’t want to be torquing on the mirror modules that are 70 kilograms each while you’re on a boom that is flexible,” Ferrie explains.
New detector technology
In the 1960s and early ’70s, Weisskopf and fellow scientists at Columbia University learned valuable lessons about the challenges to come.
In 1968, shortly before Weisskopf arrived, Columbia scientists sent their first attempt at a detector to the fringes of space on a sounding rocket. If all went as planned over the span of five minutes, the polarimeter would reflect incoming X-ray photons off lithium blocks into surrounding small chambers known as gas proportional counters. When a gas molecule in a counter absorbed a photon, the molecule would eject an electron. By counting the electrons, and by extension the photons, the scientists would determine which counter received the most photons and from that calculate the direction of polarization.
Disappointingly, the instrument delivered no detections, so the scientists made a major adjustment for the second flight two years later. They added four additional polarimeters, each with graphite crystals that served the same function as the lithium blocks in the fifth polarimeter. The strategy worked: The team gathered X-ray polarimetry about a then-newly discovered pulsar 7,000 light years away, rotating 30 times a second at the center of the Crab Nebula, a supernova remnant and a bright source of X-rays.
Nevertheless, Weisskopf recognized the limitations of the photon-counting method. He came to realize that to make a bigger impact on astronomy, an X-ray polarimeter in space would need to create focused images.
Enter scientists from Italy’s National Institute for Astrophysics and National Institute for Nuclear Physics and France’s Institute of Nuclear Physics. After working with members of the Italian team on other projects, Weisskopf learned in 2000 that they had invented just such an X-ray detector relying on the photoelectric effect. In the concept, X-rays crossed a beryllium window and interacted with a layer of dimethyl ether gas whose molecules, when they absorbed an X-ray photon, ejected an electron like the old gas counters did. The electron traveled in the direction of polarization, ionizing more gas molecules along the way. These are the tracks that IXPE will image.
Weisskopf teamed up with the Italian scientists, and together with Ball Aerospace they developed the pitch that NASA accepted in 2017. With Italy providing the dimethyl ether gas detectors, Marshall took responsibility for building IXPE’s three cylindrical telescopes, and Ball Aerospace agreed to design and build the spacecraft body and to put all the elements together and perform testing.
Final jolt
Last year, IXPE officials received a second surprise, and this one was less pleasant than finding out a larger launch vehicle was available. The rounded, triangular aluminum deck that must hold IXPE’s telescopes steady arrived warped from a vendor that Ball Aerospace said it won’t name as a courtesy to the vendor.
Ball normally would have machined that part in house out of a solid block of aluminum, but the part was just a little too big for Ball’s facility.
To evenly line up the telescopes with the detectors, the top of the structure needed to be even so the telescopes would be the same distance from their respective detectors.
“The whole structure was, like, warped,” Ferrie says. “It looked like if you fried a potato chip.” That wasn’t going to work: “You want each mirror module” — aka telescope — “to be sitting on the surface at the same height.”
The order had already taken eight weeks, with only a couple of weeks left in the schedule margin until the lack of the part would delay everything.
“‘It’s going to affect launch,’” Ferrie remembers thinking. “It was a huge deal.”
A group from Ball flew out to the vendor.
“We had a lot of discussions over, ‘OK, what was your machine speed — what would help?’” Machining away aluminum from a solid block at a fast speed, to create a thin wall, can contribute to potato chipping, Ferrie says.
Together the teams redesigned the structure to have ribbing on the back to provide support. They slowed down the machine speed and heat-treated the metal, subjecting it to hot and cold temperatures, earlier in the machining process to make it stiffer sooner, and they did that twice instead of once. They machined pockets out of the metal to make up for the added thickness of the ribs.
“Our machinists here and their machinists,” says Ferrie, referring to the vendor, “along with our designers, came up with an amazing product that is actually lighter in the end.”
Her priority now until the launch: “Finishing the test campaign,” Ferrie says. IXPE is about science and tax dollars and international collaboration. But it’s also about achieving the vision of one very persistent scientist.
“We kind of always joke Martin has been waiting 70 years for this,” Ferrie says, exaggerating the 50-plus years since Weisskopf took part in the sounding rocket launches.
The waiting may end as soon as November, the earliest IXPE could be launched.
Related Topics
Space Science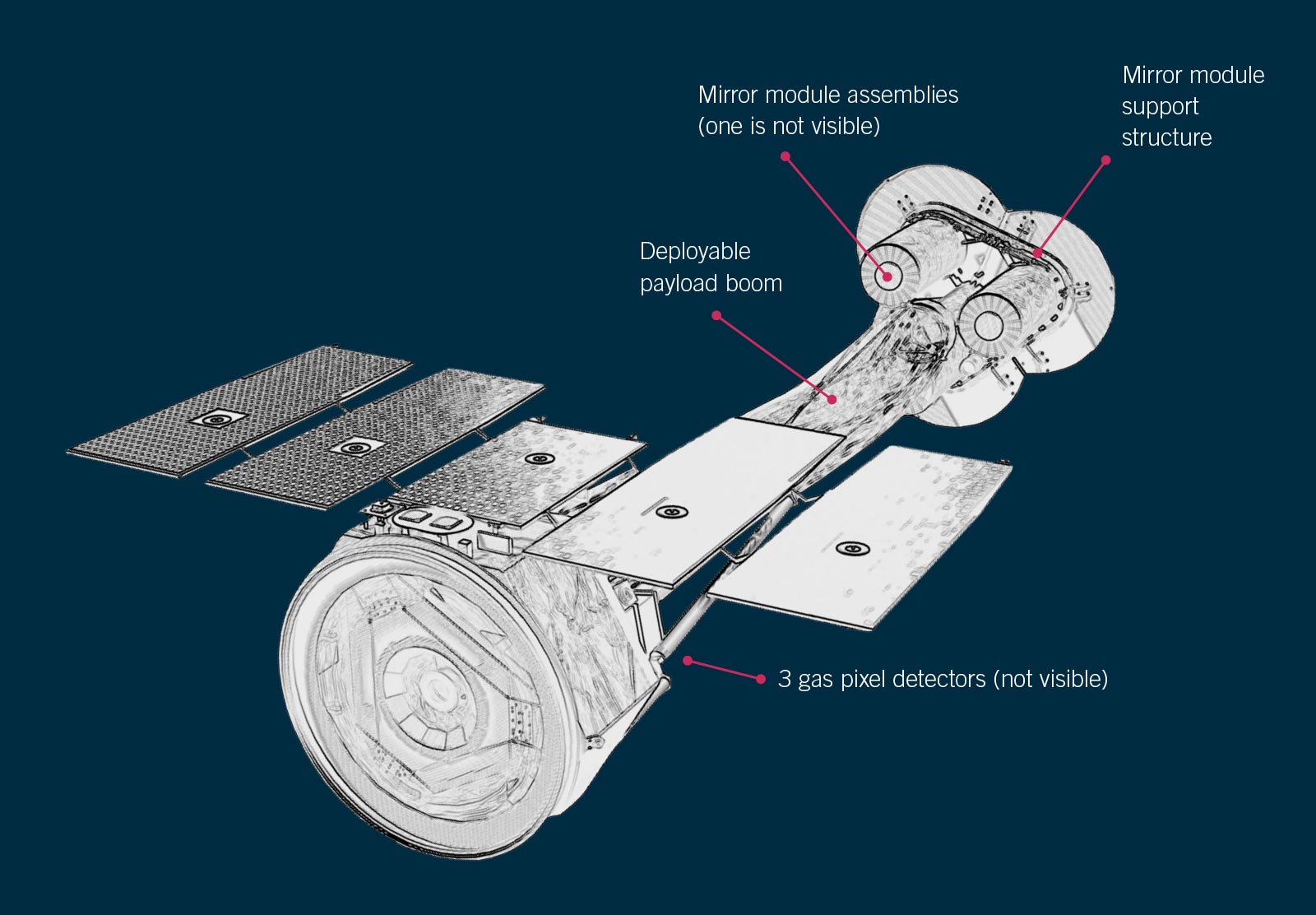
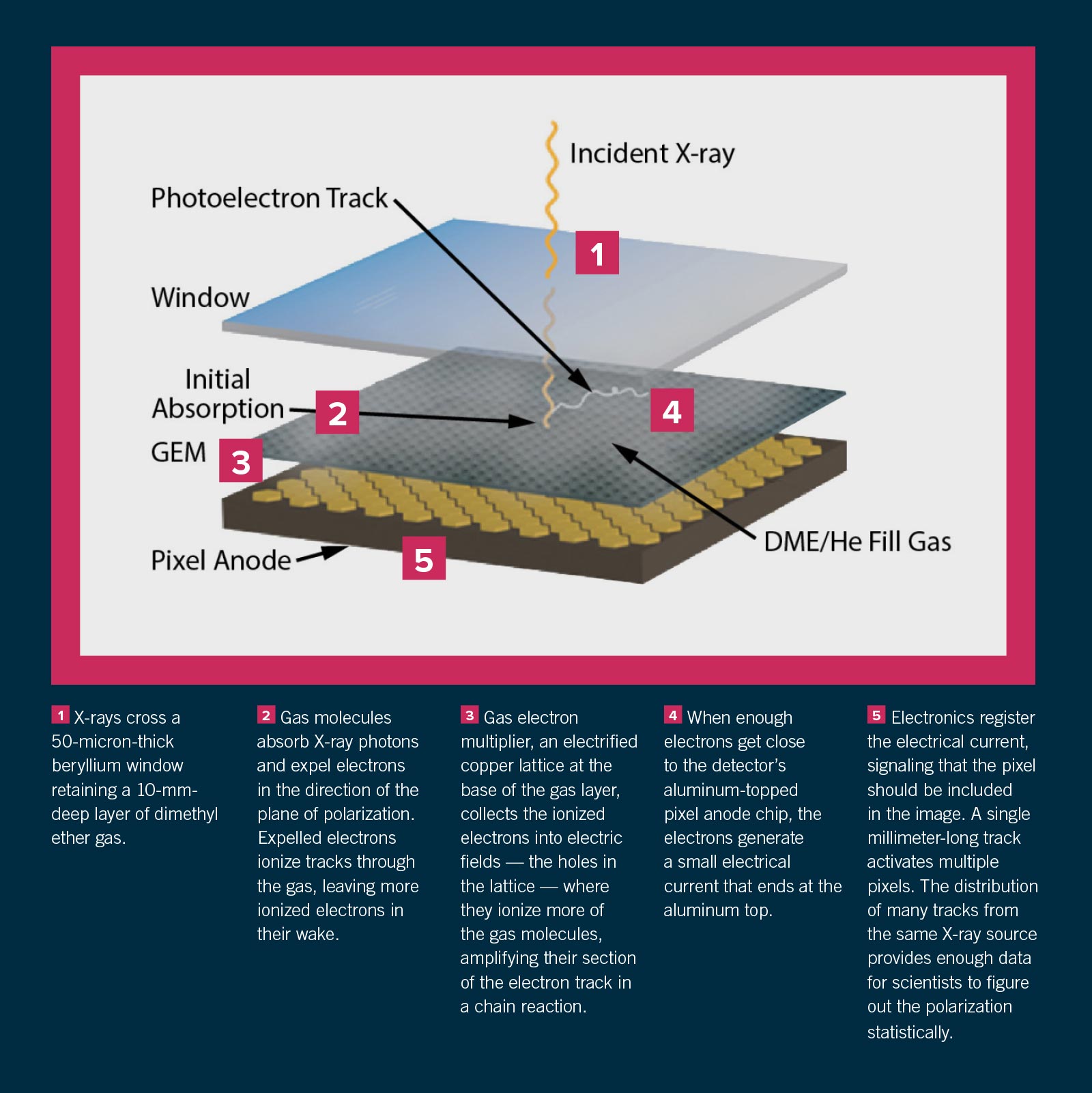