Stay Up to Date
Submit your email address to receive the latest industry and Aerospace America news.
The Astrodynamics Technical Committee advances the science of trajectory determination, prediction and adjustment as well as spacecraft navigation and attitude determination.
Observations by Johannes Kepler 400 years ago showed that the orbit of Mars is not exactly circular, as was widely assumed, but elliptical. Later, Isaac Newton used his law of gravity to prove that all repeating orbits must be ellipses, but in nature, this is a mathematical abstraction. This realization provides opportunities for mission planners.
A case in point is the James Webb Space Telescope. In January, a final post-launch correction burn sent Webb into a halo orbit, meaning an elliptical one, around the second Lagrange Point, L2, a gravitational equilibrium point created by the gravity of the sun and Earth. Here, the telescope can orbit with little expenditure of propellant, its cold-side optics aimed away from the sun to deliver what should be many years of observations.
From an astrodynamics perspective, a major challenge was getting Webb into this final orbit without overshooting L2. A braking maneuver was not an option, because that would have required imparting force opposite to the direction of its motion away from the sun, an impossibility given that the telescope’s thrusters had to be located on the sunward side to avoid interfering with its supercooled instruments. The solution to this conundrum was to launch Webb with slightly less velocity than was needed to reach L2. Three burns were conducted, including the final one in January, to gradually increase its speed by just the required amount. This was accomplished so efficiently that the remaining fuel budget should extend the mission for many years beyond its five-year design life.
Another useful gravitational complexity comes from the nonspherical shape of Earth and the consequent irregularities of the geopotential field. When SpaceX Starlink satellites are first deployed, mission planners use Earth’s natural gravitational perturbations to change their ascending nodes with no direct fuel cost. To spread 60 Starlink satellites into three orbital planes, the altitudes of a group of 20 satellites are raised by electric propulsion. The satellites in this group drift relative to the others for about a month to reach the desired difference in ascending node. The process is then repeated with two more to achieve an orbital plane spacing of roughly 20 degrees for each group. As of October, SpaceX was up to 3,133 working Starlinks, according to Jonathan’s Space Report, on its way to building a final constellation of about 12,000 satellites.
NASA’s Cislunar Autonomous Positioning System Technology Operations and Navigation Experiment provides another striking example of complex mission design. In November, CAPSTONE arrived at its near- rectilinear halo orbit, or NRHO, according to Advanced Space of Colorado, NASA’s mission partner for CAPSTONE. With the cubesat experiment, NASA plans to learn how to operate in this orbit before the lunar Gateway space station is sent there. The NRHO has many advantages. The path is so eccentric that CAPSTONE, and later Gateway, will pass well into the region where Earth’s gravity has significant effects. This means that a spacecraft bound for the Gateway will have low acceleration requirements. Also, the orbit affords constant line of sight to Earth for communications. The astrodynamicists who designed CAPSTONE’s trajectory made clever use of a ballistic lunar transfer orbit with very low fuel requirements. The CAPSTONE team endured a scare in September, when during one of the trajectory maneuvers toward NRHO, the satellite began to spin, and an “operational emergency” was declared. Three-axis control was regained in October, Advanced Space and NASA said. A valve malfunction is the suspected cause of the spinning.
Stay Up to Date
Submit your email address to receive the latest industry and Aerospace America news.
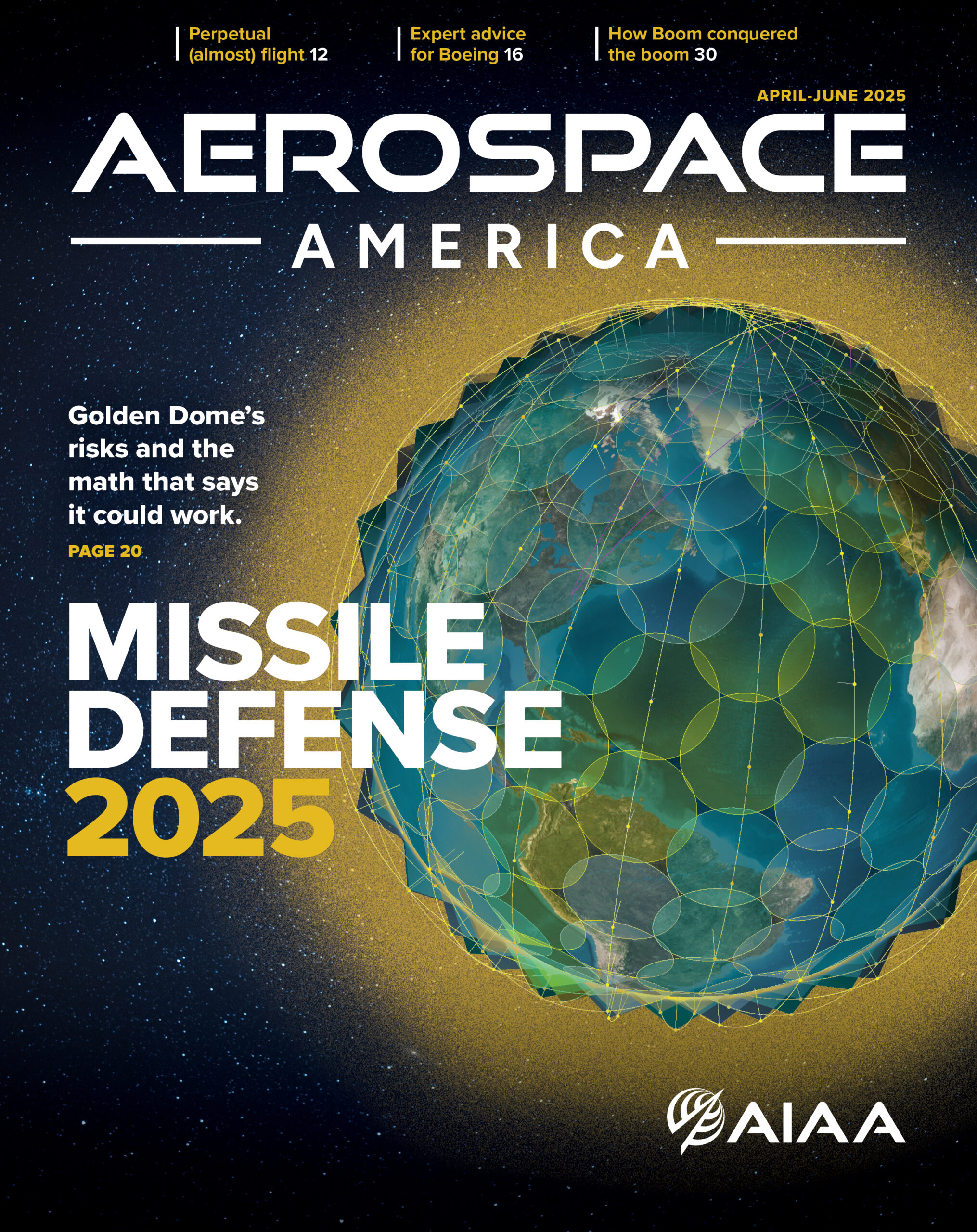