Stay Up to Date
Submit your email address to receive the latest industry and Aerospace America news.
Mission planners are now fully coming to grips with the twin hazards posed to astronauts by long durations in weightlessness and exposure to cosmic radiation. Scientists and engineers are working on faster propulsion technologies to cut down on trip time, as well as a suite of countermeasures, aimed at bringing the red planet safely within human reach.
In the movies, at least, it’s when the astronauts actually land on Mars that the trouble starts, vis-à-vis monstrous aliens or a dust storm like the one that stranded “The Martian” Mark Watney. In real life, astronauts will face some of their greatest peril during the journey itself.
The reason is the likely transit time. If propelled by conventional chemical rockets, and depending on the trajectory, a round-trip mission to the red planet including time on the surface could take as long as 900 days. That would mean spending about twice as many consecutive days in weightlessness as the all-time record holder, Russian cosmonaut Valeri Vladimirovich Polyakov, and nearly three times more days than the U.S. record holder, astronaut Scott Kelly.
The surface of Mars, where gravity is about 40 percent of Earth’s, would offer a slight respite from total weightlessness but perhaps not a medically significant one. “What does 0.38g mean for us? To just assume that [gravitational] loading is sufficient to preserve health, we don’t know that,” says former astronaut Jim Pawelczyk, a Penn State associate professor of physiology and kinesiology who has served on the NASA Advisory Council.
Once outside of Earth’s protective magnetosphere, the real trouble would begin. Galactic cosmic rays — fragments of atoms flung in all directions by exploding stars and other celestial events — would penetrate their spacecraft hull and tear through human tissue, causing illnesses in the short term and damaging DNA for longer-term consequences. These rays would be even harder to shield against than the solar energetic particles blasted out by our sun from time to time. No one really knows what the long-term effects will be beyond the magnetosphere, because the Apollo crews spent less than two weeks outside this shield.
By the time a crew arrives at Mars, each member could be ill from radiation and weak, having lost as much as 40 to 50 percent of his or her musculoskeletal strength. The crew members might be in little shape to effectively explore Mars, and if they make it home, serious health issues could plague them the rest of their lives.
Is there a silver bullet that might save humanity’s first mission to Mars? Possibly: “The shorter you can make the mission, the safer we feel like it could be for the astronauts,” says Damon Landau, an outer planet mission analyst at JPL, the NASA-funded Jet Propulsion Laboratory in Pasadena, California.
Radiation data from Mars-bound spacecraft, such as the Curiosity rover and its cruise stage, suggest that a crew must get out and back in 200 days, explains Dennis Bushnell, chief scientist at NASA’s Langley Research Center in Virginia.
That transit time would be impossible with existing propulsion technology, so NASA knows it needs a game-changing propulsion technology, probably of the nuclear variety, and work is underway to deliver it. The conquest of Mars also would depend on lessons learned in a re-conquest of the moon. NASA wants to get astronauts back there in the 2020s for the first time since “Papa Was a Rollin’ Stone” topped the charts in December 1972.
A Lunar Orbital Platform-Gateway would be assembled in orbit around the moon as a base for astronauts. “The gateway is a great opportunity to test the system, reduce the risks, and make sure we understand how we’re going to protect the crews and keep them safe,” says John Baker, manager of the Human and Robotic Mission Systems Architecture Office at JPL.
Once on the surface of Mars, the radiation exposure would be a third less potent per day than in space, according to measurements from the Curiosity rover. Still, the crew would have to protect itself in a shielded habitat, perhaps setting up base within the subsurface caverns called lava tubes that are open in places to the Martian air.
“On planet, the body needs some 4 meters of regolith or ice” as shielding, says Bushnell. “Reality is different from the cartoons of folks wandering around Mars.”
With Mars offering a degree of safe harbor, then, it’s the open ocean of space that mission planners must deal with.
Are we there yet?
Part of the trick to compressing the journey would be launch at the right time. As the planets move throughout their orbits, an ideal alignment window for a launch from Earth to Mars occurs every 26 months. A long-stay, conjunction-class trajectory during this window carries the lowest energy needs for propulsion, and thus mass that must be expensively and prohibitively launched off Earth as well as carried along to Mars. This trajectory lobs the spacecraft into a rendezvous with Mars as the planet swings toward the opposite side of the sun from Earth (called a “conjunction” in astronomy) relative to Earth’s location at launch. The return trip does the same in reverse, spending a few hundred days on Mars until an optimal alignment for meeting Earth in conjunction. A conventional, chemical propulsion-based conjunction mission would therefore run 900 or so days, with 240-some days spent on each interplanetary transit leg.
So-called opposition-class trajectories, on the other hand, typically take advantage of this alignment for only one leg of the voyage. The rendezvous with Mars or with Earth occurs, relative to launch, when the planets end up on the same side of the sun, termed an opposition in astronomy. These missions have greater timing flexibility, but higher propulsion requirements to more brute-force the spacecraft’s planetary rendezvous. To gain speed on the trip’s long leg, the crewed spacecraft can actually fall inward into the solar system, completing a flyby of Venus for a gravity assist.
Overall, opposition trajectory-based missions cut down on off-Earth durations, running perhaps half as long — 450 days — as conjunction missions, though with more time spent in space instead of on the Martian surface, where expeditions can last as little as a month.
New paths to Mars
To understand why enthusiasm for nuclear propulsion is growing, it’s worth looking at other technologies that might have a role in a compressed mission, but a supporting one. Solar electric propulsion, in which electricity generated from sunlight usually ionizes a gas, such as xenon, that is shot out a nozzle to produce thrust, offers superior specific impulse, a measure of fuel efficiency, compared to chemical propulsion, because of the incredible exhaust speeds. However, the thrust is much lower because of the low amounts of mass streaming out of the engine. Getting a substantial mass up to high speeds, at least with today’s versions, would require long acceleration periods or very high available power.
While solar electric should work well for short-haul moon missions, planners do not see it sufficing for relatively massive, long-haul crewed vehicles to Mars any time soon, especially given the drop-off of solar intensity heading away from Earth’s vicinity. “With solar electric power, the arrays just become so enormous, it’s just not feasible,” says Ronald Litchford, the principal technologist for propulsion at NASA’s Marshall Space Flight Center in Alabama.
Instead, planners foresee pre-positioning mission elements, such as a lander, at Mars with the technology. “For crewed [Mars] missions, solar electric propulsion isn’t quite practical yet,” says Baker, “but using it for cargo missions is a great idea.”
“We really have to get to nuclear if we want to go super-fast,” says Litchford.
In the high-thrust nuclear variety, nuclear thermal propulsion, a material such as liquid hydrogen is heated up by the reactor, expanding out a nozzle to generate thrust. Based on NASA’s Nuclear Engine for Rocket Vehicle Application, or NERVA, program, which during the 1960s built and tested numerous nuclear thermal reactors and rockets yet was canceled by Congress in 1972 before attaining flight, the specific impulse generated is known to be about 900 seconds — twice that of typical chemical rockets.
Reducing Earth-Mars transit times has reinvigorated interest in nuclear thermal propulsion. In August 2017, NASA awarded a three-year, $18.8 million contract to Lynchburg, Virginia-based BWXT Nuclear Energy Inc. to lead a new program of reactor design and fuel fabrication. BWXT is working closely with Aerojet Rocketdyne, headquartered in Sacramento, California, as it pursues engine and vehicle system development activities through a NASA Research and Technologies for Aerospace Propulsion Systems 2 contract. The goal, says Aerojet Rocketdyne, is to build a prototype system by the mid-2020s fueled by low-enriched, instead of previous efforts’ highly enriched, uranium as fuel. Doing so would substantially lower production costs, as well as easing security and handling regulations in place to prevent capture of the weapons-grade material, or a hazmat accident.
In nuclear’s low-thrust version, nuclear electric propulsion, a fission reactor cranks out electricity to power an electric thruster. NASA has flown a fission reactor in space only once, back in 1965. But recent progress on a project called Kilopower — covered in Aerospace America’s October 2016 issue — could end that cold streak. Kilopower is a purposefully conservative project based on tried-and-true technologies, and given its weight and low power, is geared more for providing power to surface habitats on the moon or Mars. Yet it could help open the door to lighter, spaceborne reactors in the necessary megawatt-range for fast Mars transits. “One of the real benefits of Kilopower is it’s a way for us to get through all the regulatory processes and break through that barrier of not having flown a reactor in a long time,” says Litchford.
Parallel progress is continuing, with NASA funding, on electric thrusters that could be scaled to handle the desired power levels promised by nuclear electric propulsion. One kind, already deployed with solar electric systems, is the Hall thruster. Within it, electrons trapped in a magnetic field ionize a propellant, oftentimes xenon; the ions then shoot out into space to produce thrust. Another thruster type, called FRC for field reversed configuration, relies on rotating magnetic fields to isolate chunks of plasma in a cavity and then expel them.
A third sort of thruster under development is the Variable Specific Impulse Magnetoplasma Rocket, acronymed VASIMR. Its principle of operation: Radio waves convert a gas (argon, xenon, or hydrogen) into a superheated plasma, which then hurtles out a magnetic nozzle at about 180,000 kilometers per hour (112,000 mph). Ad Astra, the Texas company behind VASIMR, is preparing its latest engine version, dubbed VX-200SS, for a consecutive 10-hour run at 100 kilowatts in a vacuum chamber. Doing so would establish the thruster at Technology Readiness Level 5 on NASA’s nine-point scale, indicating validation in a mission-relevant environment. Mark Carter, senior vice president of technology at Ad Astra, explains that the next step is advancing to Technology Readiness Level 6 for an in-space demonstration in 2022.
For some mission planners, VASIMR is particularly attractive, given its blend of specific impulse and thrust, though Litchford says “all three have promise.” Longer-term, because of VASIMR’s advantageously high scalability, ambitious planners have sketched out revolutionary fast transit architectures with it. Utilizing a 10- to 20-megawatt nuclear reactor, the nuclear electric propulsion system could complete the Earth-Mars and then Mars-Earth transits in 90 and 150 days, respectively. With a 200-megawatt reactor, that interplanetary commute could be slashed further to just two months each way. Assuming a Mars stay itself of two months, a 200-megawatt VASIMR mission architecture would hit the health safety goal of getting astronauts back home in under 200 days. Langley’s Bushnell, a proponent, calls VASIMR “wondrous.”
Safety is relative
Speeding up trajectories to limit weightlessness and radiation exposure will of course only be part of pulling off humanity’s first mission to Mars.
It will be an unprecedented long trek, spanning millions of kilometers in total confinement against an utterly hostile void. The psychological stress of confinement and relentless proximity to similarly stir-crazy humans will only become exacerbated by the lengthening communication delays with Earth, which can exceed 20 minutes each way. Real-time conversation — and thus to an extent a connection — with the home world would be severed. “Even with what we call ‘fast transit,’ it’s still a severe environment and it’s still severe stress on the astronauts,” says Litchford.
NASA’s current focus on getting humans out of low Earth orbit and returning to cislunar space could help mature many of the technologies needed to get to Mars faster and sustainably for crews’ sake. This moon-to-Mars approach, stipulated in Space Policy Directive 1 issued last December, would use NASA’s new Space Launch System and commercial space vehicles to establish the Lunar Orbital Platform-Gateway to support human and robotic missions in the 2020s and beyond.
“The first step is the gateway station,” Landau says. “Something where we have the crew in deep space far away enough from Earth where the environment is similar to what you might see on a mission to Mars.”
If breakthrough propulsions systems continue to elude, however, the conversation could shift to the broader ethical question of the acceptable risk to which a government agency, such as NASA, can allow citizens to voluntarily submit. Even if misery and death were all but guaranteed, volunteers would still eagerly sign up for a historic voyage to the red planet. “That’s human nature to say, ‘Gosh, I really want to be that first person on Mars,’” says Penn State’s Pawelczyk.
The burgeoning commercial space sector might just beat the legacy astronautics agencies to the punch. SpaceX’s Elon Musk announced in September 2018 the first private passenger trip around the moon slated for 2023, and the company still intends to send both cargo and crew to Mars just one year after, enabled by the amusingly named BFR vehicle, short for either Big “Falcon” Rocket or another F word.
Whether participants in this style of Mars mission would merely need to sign a waiver acknowledging the health risks will remain to be seen. But NASA and the American public will have to decide how much beyond established well-being thresholds we should all be willing to go in our pursuit of destiny at Mars.
“How safe is ‘safe’?” Pawelczyk asks. Ultimately, he says, the issue of astronaut safety is “as hard as we choose to make it.”
“On planet, the body needs some 4 meters of regolith or ice” as shielding. “Reality is different from the cartoons of folks wandering around Mars.”
Dennis Bushnell, chief scientist at NASA’s Langley Research Center

About Adam Hadhazy
Adam writes about astrophysics and technology. His work has appeared in Discover and New Scientist magazines.
Related Posts
Stay Up to Date
Submit your email address to receive the latest industry and Aerospace America news.
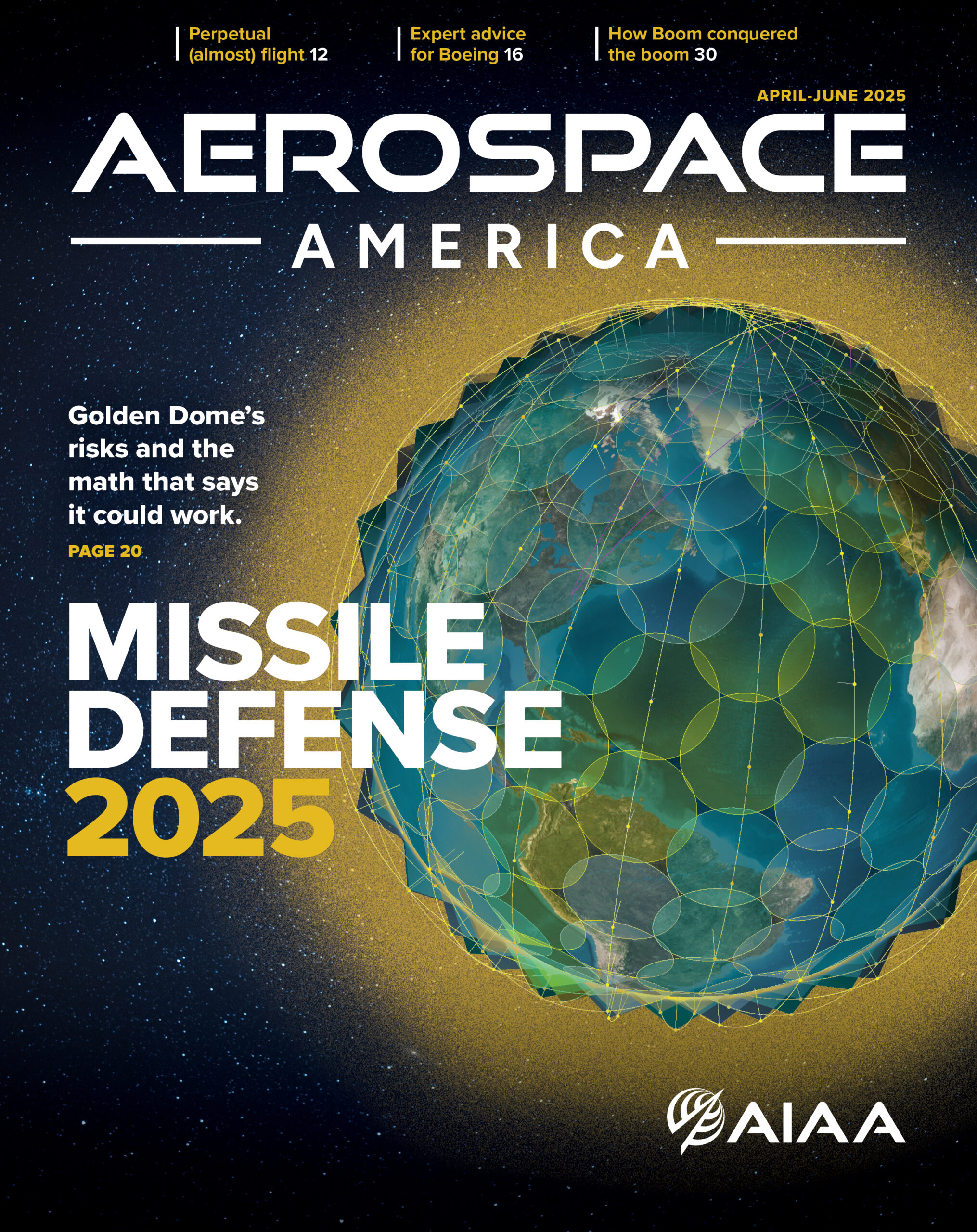
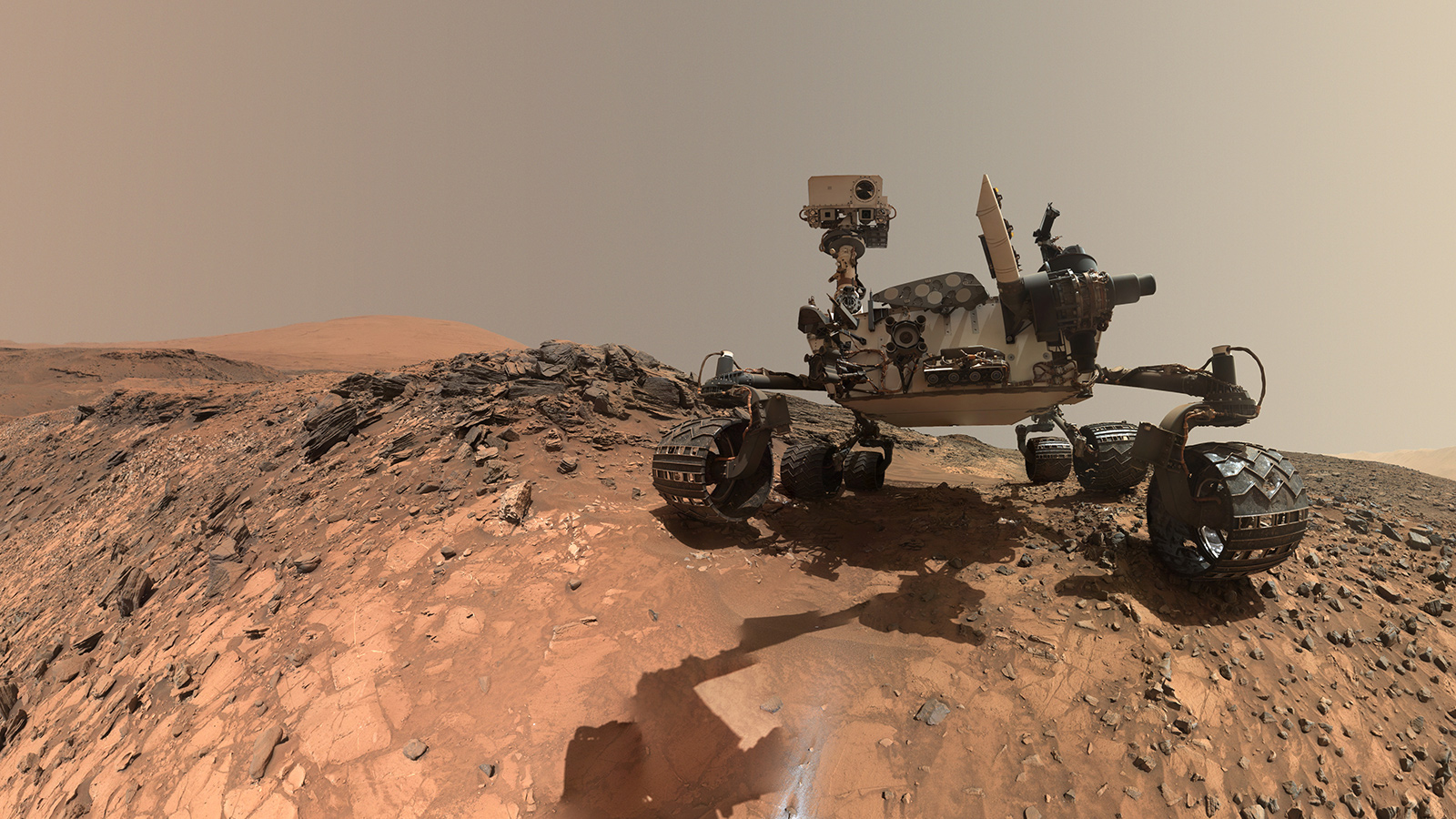
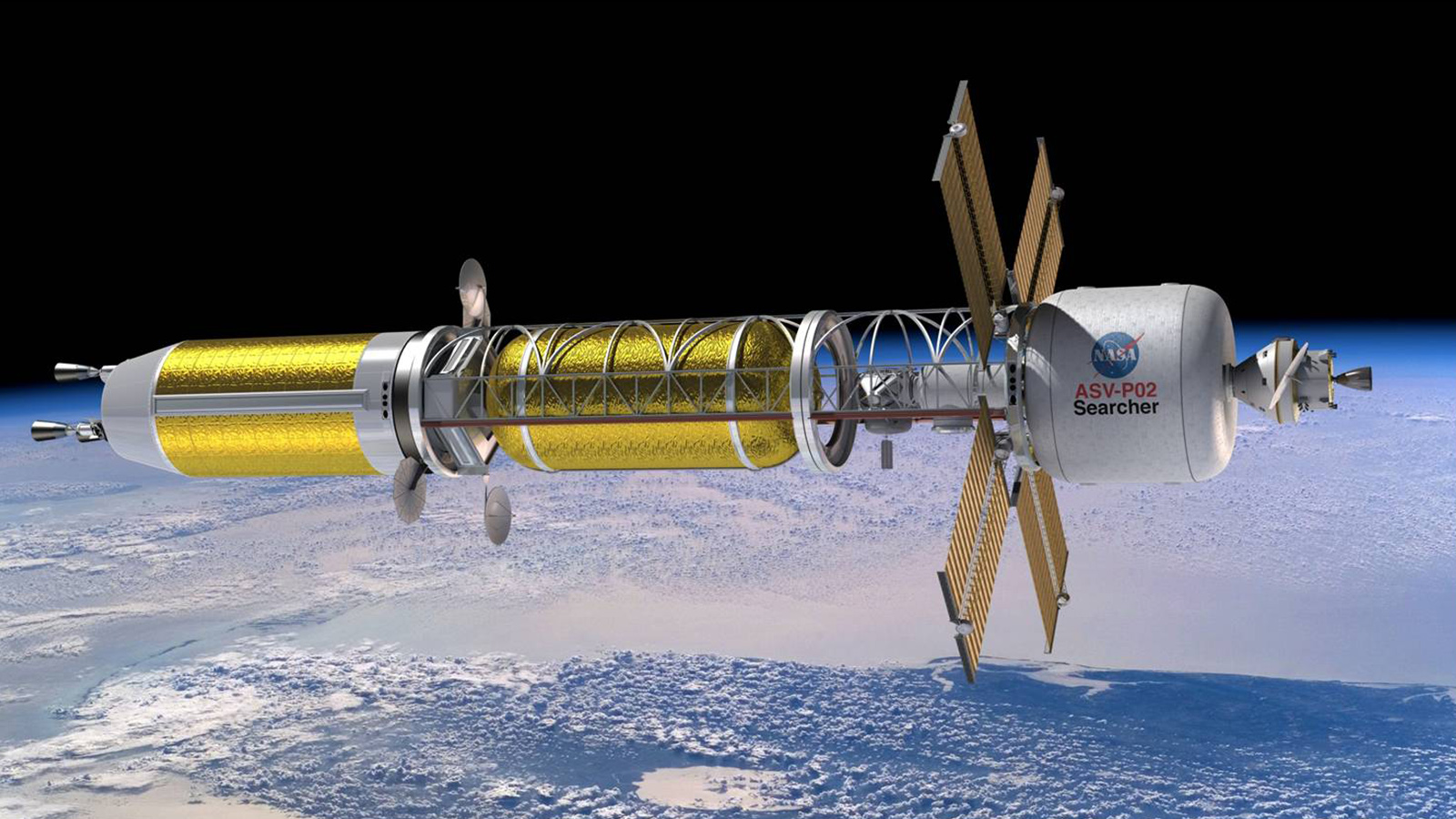
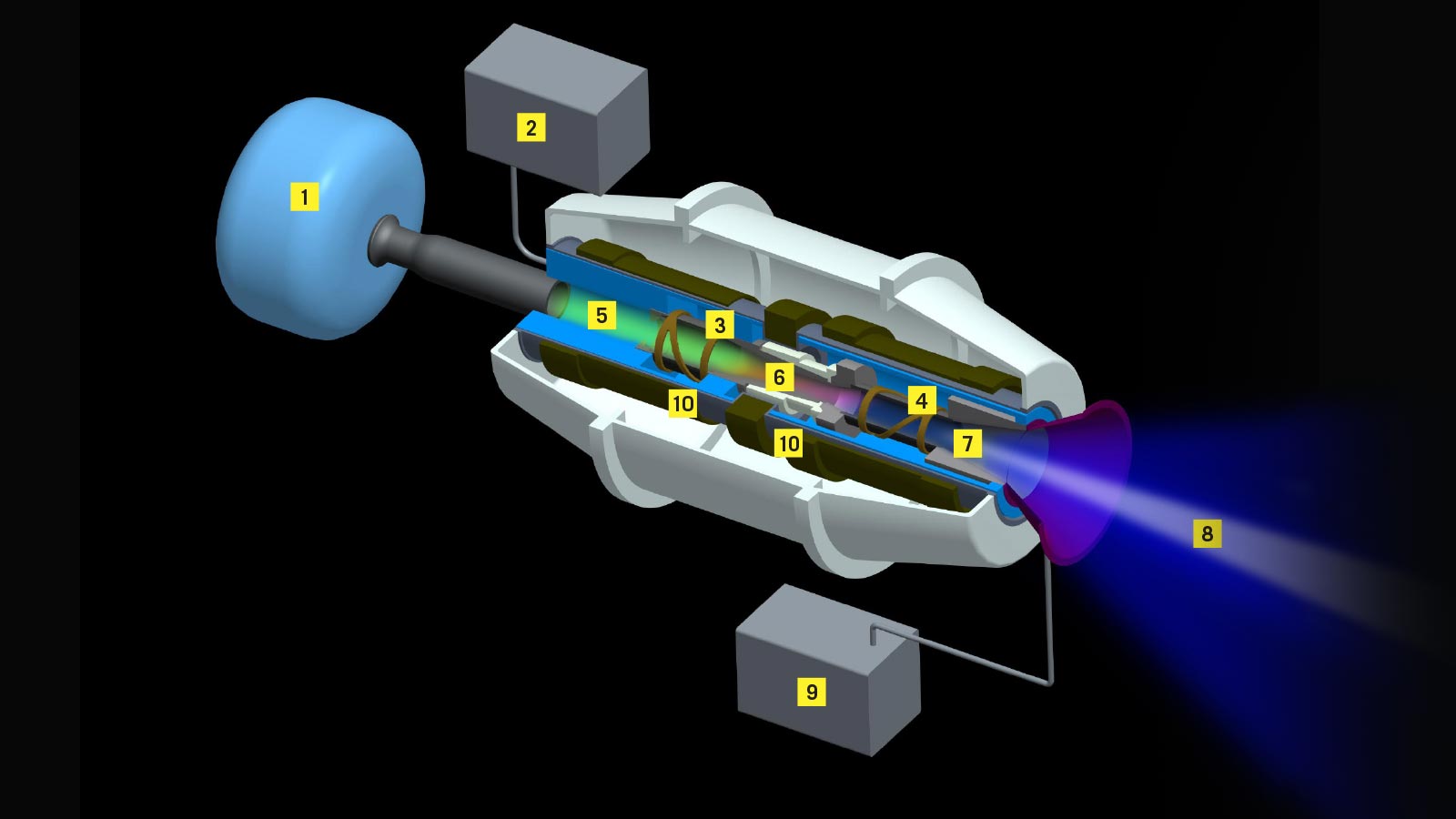