Talking (space) trash
By Adam Hadhazy|March 2019
An easily overlooked, yet critical element of long-duration lunar or Martian missions will be dealing with all of the garbage we humans inevitably produce.
The essentials of Earthly life — air, water, food, climate control — can’t be taken for granted aboard a spacecraft bound for deep space. Now receiving deserved attention for such a trip is another thing we surface-lubbers take for granted: taking out the garbage.
Over the next few decades, NASA has long-duration missions in its sights. These excursions include a lunar gateway station in cislunar space, as well as pursuing a yearslong crewed voyage to Mars. Both missions will take astronauts away from the convenient, low Earth orbit lifestyles they’ve grown accustomed to during the space shuttle and International Space Station eras. On Earth’s doorstep, trash disposal aboard the ISS is easy: Simply load up a docked resupply vehicle, cut it loose, then let it burn up during atmospheric re-entry. With new vehicle-loads of fresh supplies arriving every few months, there is little need for recovering consumables, although since 2008 astronaut urine has been recovered and converted to drinking water [See “Dealing with nature’s call”].
Deep-space crews will be largely on their own, which means NASA is increasing emphasis on the three R’s of trash management: reduce, reuse, recycle. In preparing for this future, the agency announced in October that it has selected Collins Aerospace, a 70,000-person company based in Florida, and Sierra Nevada Corp., or SNC, of Colorado to develop competing trash compaction and processing systems. These systems will compress and melt down garbage, slashing rubbish volume and venting to space resulting vapors that could contaminate cabin air. That’s the “reduce” in the three R’s. The companies are also investigating ways to fulfill the other two R’s. Capturing valuable consumables, such as water and potentially useful gases, released during trash processing could enhance mission architectures and scope, allowing for more focus on science instead of crude provisioning.
“We want to ‘close the loop’ on resources like water and air as much as possible. That’ll help us reduce our reliance on resupply,” says Shawn Macleod, senior business manager for space systems at Collins Aerospace.
By early 2020, both companies will have completed an initial phase of research and development of their trash compaction and processing systems, for which NASA plans to award between $500,000 and $1 million to each. A preliminary design review will follow, after which a second phase lasting around two years will see the initially satisfactory system (or systems) matured to flight readiness for a technology demonstration on the ISS.
These kinds of systems could deliver benefits beyond keeping trash in check and compensating for severed supply chains. The final solid waste products from both Collins’ and SNC’s systems are hard, flat tiles. Primarily made of melted, compacted plastic, these dense tiles could double as radiation shielding to mitigate this major hazard of deep-space travel. Any mission outside of Earth’s protective magnetosphere, to the moon and beyond, subjects its crew to solar and cosmic particle bombardment that can cause radiation sickness, increased cancer risks and degenerative disorders. “The idea is to repurpose the trash for something, other than just storing it somewhere,” says John Wetzel, a program manager at SNC leading the trash compaction and processing unit development.
Should a spacecraft land at Mars, rendering trash into stable, sterile tiles could also address another concern that’s always on the minds of planetary scientists. “We want to make sure with our trash that we’re not contaminating an environment with human presence,” says Macleod.
Eliminating bacteria in the smears inevitably left inside of astronauts’ food pouches, for instance, will cut down on the risks of Earthly microorganisms infiltrating pristine, extraterrestrial locales where we humans hope to detect signs of alien life. The system could help assure planetary protection.
Rubbish routines
Nowadays, trash management on orbit is hilariously low-tech. Station astronauts duct tape their disposables up into small bundles, dubbed “trash footballs.” These garbage pods are loaded into a Cygnus or Progress resupply vehicle — whatever happens to be docked — that is later jettisoned for incineration in Earth’s atmosphere. Voilà, waste problem solved.
This protocol, such as it is, has worked fine because of the minimal needs for resource recovery and given the amounts of trash generated — on the order of 2 kilograms per astronaut per day. Examples of what astronauts throw out include packaging, paper, tape, filters, food containers, and various personal hygiene items such as cleaning wipes, towels and dirty clothes (doing laundry on station is not an option).
Without resupply vehicles to serve as dumpsters, one potential trash tactic in deep space would be to simply chuck the waste into space via an airlock. But this comes with drawbacks, says Uday Hegde, who studies aerospace waste management and water reclamation at Case Western Reserve University in Cleveland and who is not involved in either the Collins or SNC projects.
For starters, in space — just as on Earth — practical trash container bags inevitably leak. “None of these bags are totally impermeable,” says Hegde. “There is always some leakage of gases and vapors.” The problem is compounded in the airlock disposal scenario. The low pressure generated when pumping air out prior to evacuation pulls liquids and gases out of solid waste. These volatiles can then freeze onto airlock surfaces, eventually interfering with proper hatch closures. Furthermore, upon repressurization and thawing, the atmosphere in the airlock would need to be filtered so the gases and vapors do not contaminate the spacecraft cabin’s air.
In addition, loading and venting the airlock, or alternatively doing a cubesat-style launch also via the airlock, are astronaut-labor-intensive. Hegde and colleagues have calculated how many trash footballs, along with the number of disposal events, would be needed to stay on top of trash management for gateway and Mars expeditions. Consider a 180-day mission, such as a stay aboard the gateway or the trip to Mars, with a four-person crew. The crew would generate about 3,000 trash footballs requiring at least 800 disposal events. Expand the crew size to six and the duration by another month, and the figures rise to 5,000 trash footballs and at least 1,200 disposal events. “You don’t want to be constantly doing these operations, taking up astronaut time,” says Hegde.
Furthermore, although littering the vastness of interplanetary space all the while on the way to Mars would not be such a big deal — the chances of another Mars-bound craft ever encountering the debris would be infinitesimal, says Hegde — accumulating garbage in the vicinity of the gateway could begin to pose a “space junk” problem, already increasingly of concern in Earth orbit.
Trash disposal reimagined
The trash management systems by Collins and SNC attack these myriad problems with a broadly similar approach, known as heat melt compaction. Each concept is centered on a sealable chamber. The astronauts would “put the trash inside, close the door, hit ‘go,’ and the whole process is automated,” says SNC’s Wetzel. The task is meant to be simple and far less labor-intensive for busy astronauts than chucking garbage into space.
Over a period of hours, low pressure and heat, followed by compression, remove water and other volatiles while reducing the trash’s volume. The end result is a solid, flat tile about an inch thick and shaped as wide and deep as the chamber, which in early designs has ranged from 9 to 16 inches square. Both systems are intended to reduce the trash volume by over 90 percent, with percentages in the 70s-to-80s range for air and water content recovery.
This trash management tactic was initially explored by engineers at NASA’s Ames Research Center in California. Orbital Technologies Corp., a Madison, Wisconsin-based company acquired by SNC in 2014, worked closely with Ames on these initial efforts under a NASA Small Business Innovation Research program. The NASA test unit developed many of the technologies underlying the approach SNC and Collins are now building upon in their own projects. “We want to take all the lessons learned by NASA,” says Macleod, “to mature our trash compactor processing system and make sure it’s integrated with the ISS [for the demonstration] as seamlessly as possible.”
Collins is further drawing upon experience in designing trash compactors for commercial aviation, for instance in service aboard the Airbus A350 XWB airliner. Collins Aerospace was formed in November 2018, when United Technologies of Connecticut acquired Rockwell Collins, combining it with UTC Aerospace Systems, known in the industry as UTAS. Those companies brought in experience in human waste management as well, again for aviation, but also in space. Collins is under contract by NASA to construct the next-generation space toilet, dubbed the Universal Waste Management System [see sidebar].
Heat melt compaction in action
In appearance, SNC’s system looks somewhat like a dorm room-style mini-fridge, while Collins’ is more juicer-like. Within each system’s chamber, heat melt compaction proceeds as follows. After astronauts pop refuse into the chamber and seal it, the next phase is air and water recovery. The pressures in the chambers plunge below a tenth of normal atmospheric pressure, which at sea level is 101.325 kilopascals, suctioning air and water out of the trash bag. Macleod describes the Collins system as using a relatively low temperature at first for drying, around 60 degrees Celsius. “What we’re trying to do is get as much of that air and water out before we start really heating things up and getting contaminants — the nasty stuff from boiling and melting plastic, like nitrile gloves,” Macleod says.
When air and water recovery is mostly completed, the temperature rises and compression kicks in. The SNC system reaches about 150 degrees Celsius. “The goal is to encapsulate trash and defeat microbes,” says Wetzel.
The means of physical compression is a key difference between the companies’ systems. In SNC’s concept, an air-inflated bellows creates a compression pressure that pushes a piston-driven panel through the chamber to mash the trash against an interior wall. By contrast, Collins will move its piston and panel with a linear screw drive mechanism, a common type of electric actuator that appears in applications from DVD drives to aircraft doors. Both these approaches diverge from the NASA unit, which employed a scissors lift mechanism consisting of crisscrossing members that could extend or retract. You’ve probably seen scissors lifts at work here on Earth. They commonly elevate aerial work platforms for people to change signs and light bulbs, for instance.
Wetzel and Macleod agree that bellows and linear screw drives have fewer moving parts than a scissors lift, offering better potential reliability. “One of NASA’s figures of merit, or hot buttons, is reliability,” says Macleod. “On a long-duration mission, they can’t afford for the compactor to break down, and the more moving parts you have, the more susceptible you are to reliability issues.”
Also, in terms of reliability, a problem that has plagued early iterations of heat melt compaction systems is clogging of the vent ports. At this early stage of development, Collins is pursuing a modular design, moving components around to optimize performance and curtail clogging issues. “We’ve got vent ports all over the place on this thing and all sorts of different areas where we have heaters that we can turn on and off,” Macleod says.
All told, trash compaction and processing cycles for the preliminary systems are slated to last approximately six hours, allowing astronauts to run a couple of loads per day. That cycle time might need to decrease to handle all the rubbish on moon and Mars missions.
Trash heap to trash reap
As for the critical recovery of vented gases, including water, this takes place in downstream components of the trash compaction and processing systems. Compared to the chambers, these systems are less fleshed out at this stage of the NASA solicitation.
SNC and Collins each have experience in environmental control and life support systems — dubbed ECLSS, “Ek-liss” — for aerospace. ECLSS includes breathable air, potable water, comfortable temperatures, appropriate atmospheric pressure, fire detection and suppression, and of course, waste management.
SNC has worked with Collins previously on developing ECLSS for the Dream Chaser, a spaceplane intended to start ISS cargo runs in late 2020 and that might eventually ferry crew as well. Similar work is also ongoing for Boeing’s CST-100 Starliner, which is slated to take astronauts to the ISS for the first time later this year.
Collins’ ECLSS experience also extends to the ISS itself. Besides the new space toilet, the company has previously engineered oxygen-generating and water processing systems, the latter of which delivers 3 liters of potable water per day. Collins is reusing components from these well-developed systems, aeronautical and astronautical, for its trash compaction and processing. One example is a fan separator technology for aircraft toilets that sequesters liquids from gases. “We’re going to refresh that design and reuse it for application here in our trash compactor system,” says Macleod.
Many details remain to be worked out for SNC’s and Collins’ systems, and both engineering teams must for now limit themselves to ISS payload rack volume and power constraints — 500 watts in the case of the latter.
NASA hopes the fully realized systems these technology demonstrations lead to will put trash management conveniently into the background of mission activities, letting explorers worry more about exploring than taking out the trash. Indeed, with a bit of automation for storing the output tiles, the systems can be designed in a way that “would mimic what we do on Earth,” says Case Western’s Hegde, “where we set the trash out, and ‘forget’ it.”
Related Topics
Human Spaceflight“On a long-duration mission, they can’t afford for the compactor to break down, and the more moving parts you have, the more susceptible you are to reliability issues.”
Shawn Macleod, Collins Aerospace
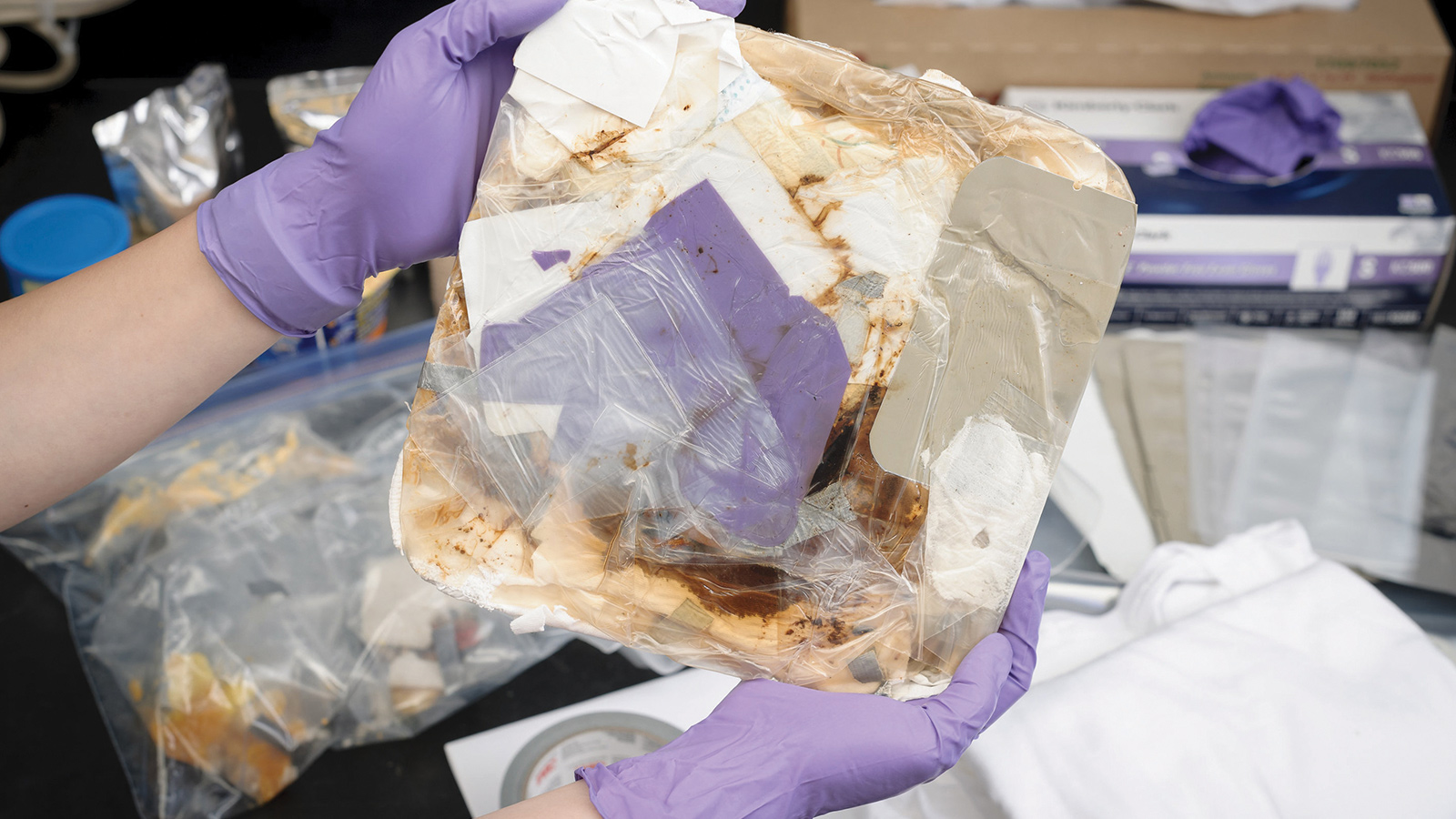
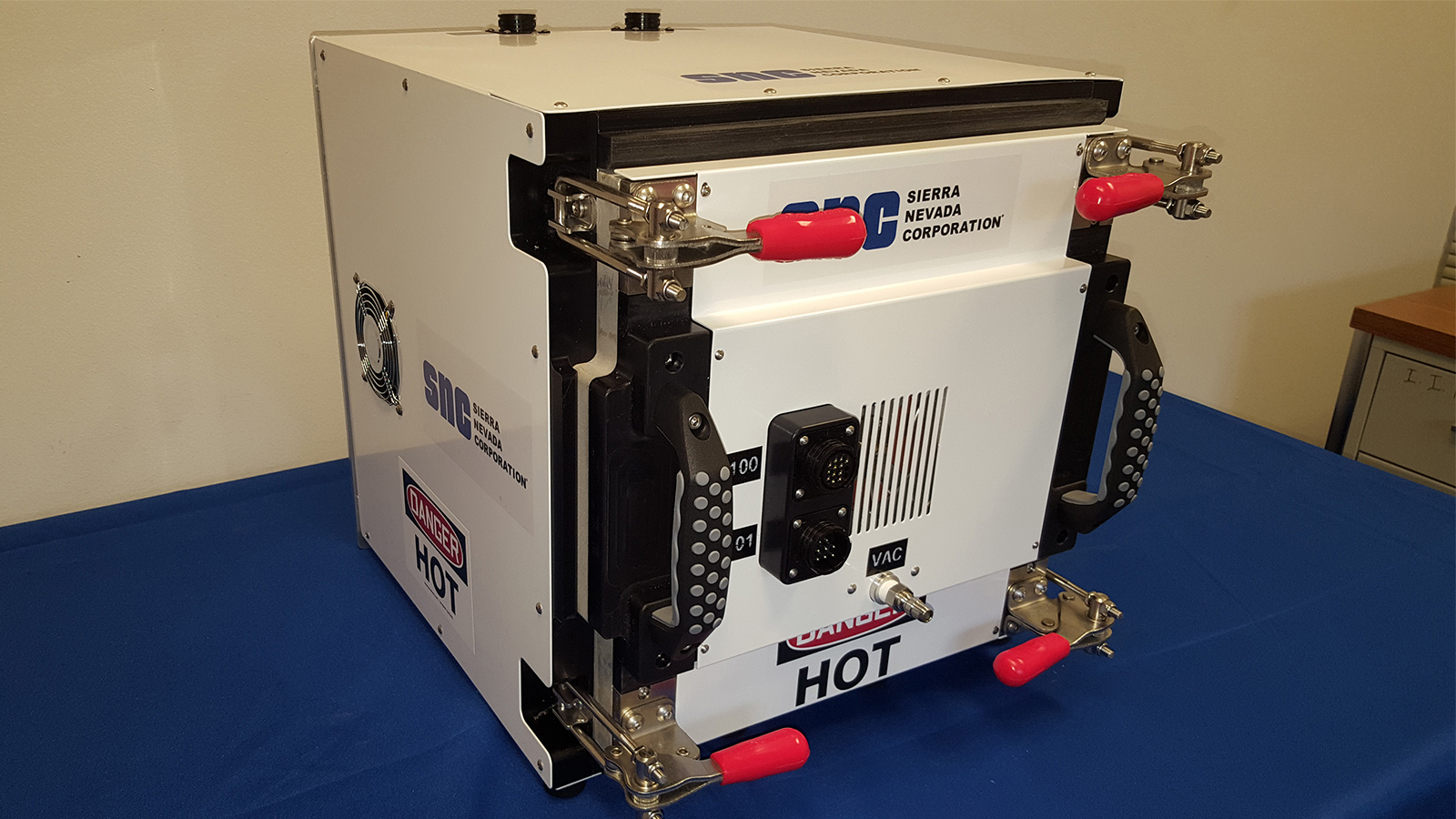
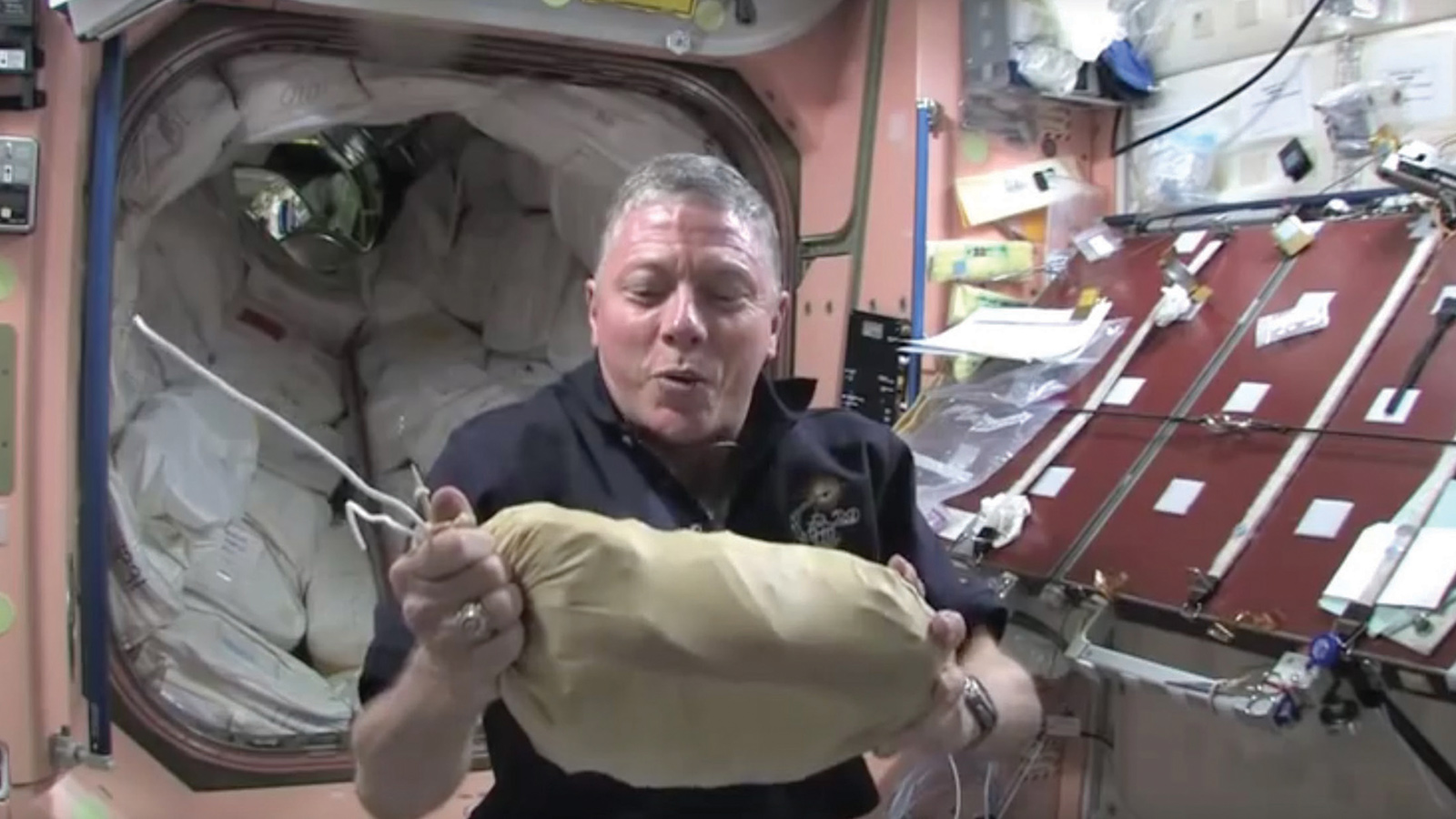
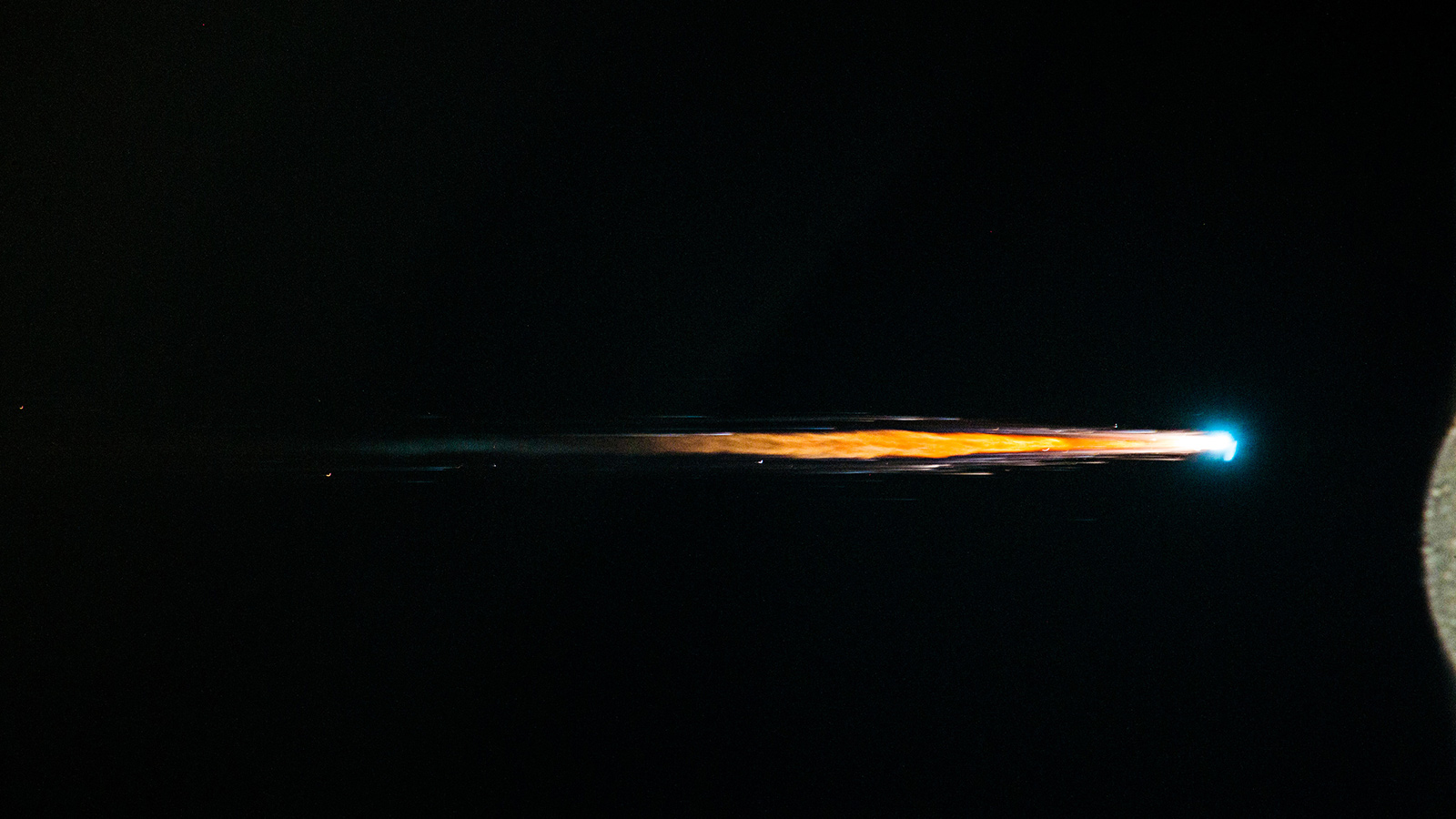