Diving deeper into the final frontier
By Adam Hadhazy|November 2021
The long-awaited start of the James Webb Space Telescope draws close, with the promise of detecting the cosmic dawn and perhaps even signs of extraterrestrial life. Adam Hadhazy explores the key science motivations and goals for what will be, if all goes as planned in December, the largest telescope yet to reach space.
Editor’s note: The sidebar in this story, “The Price of Webb,” contained an incorrect figure for the cost of operating the James Webb Space Telescope. It has been updated with the correct figure of $900 million.
Since Galileo first pointed a telescope skyward in 1608 and saw farther than any human ever had before, astronomers have strived to peer ever deeper into space and, because of what’s now known about the speed of light, back in time. Some four centuries later, hopes of continuing this quest by detecting the universe’s first light are now vested in the James Webb Space Telescope, the biggest, most powerful, most complicated, most expensive and — not unrelatedly — most delayed space telescope in history.
Motivation for the observatory traces back to astronomers realizing the limits of the Hubble Space Telescope. In the decades since its corrective optics were installed in 1993, Hubble has revolutionized astronomy and cosmology in part due to its deep-field observations involving long exposures that gather up every precious particle of light possible across a range of infrared, visible and the ultraviolet wavelengths. The first revelatory Hubble Deep Field images — gathered over a 10-day span in 1995 — showed that a tiny piece of sky, despite appearing blank and empty to the naked eye and telescopes on Earth, actually held thousands of extremely distant and therefore very young galaxies. These galaxies were primitively smaller and more disordered than modern, mature galaxies, but even so, they were clearly not the first galaxies ever formed.
No amount of observing by Hubble would ever be able to see back to the cosmic dawn, researchers accordingly fathomed. That’s because light waves from the earliest galaxies have been stretched so far into the longer infrared wavelengths that Hubble can’t detect them. Ground-based telescopes could not capture this severely stretched or redshifted light, either, because Earth’s atmosphere blocks out most infrared.
And so, ironically, “the main science goal for Webb arose from something that Hubble did not see,” says Eric Smith, NASA’s program scientist for Webb at headquarters in Washington, D.C.
As the scope of the redshift problem was becoming clear, astronomers, urged on by then-NASA Administrator Daniel Goldin, began deliberations in 1995 over the instruments and science goals for a next-generation telescope. They realized that detecting the universe’s first light, as Goldin challenged astronomers to do, would require gathering much more of the infrared range than Hubble does. Also, some of the primordial features are bound to be faint, so the new telescope’s primary mirror would need to be at least several times wider than Hubble’s. And the infrared detecting materials behind the primary mirror would need to be chilled, so that the infrared light from the earliest galaxies would stand out.
Now, after a quarter-century of work, including 17 years of construction and testing, the Webb telescope is at last ready to head to space. Careers, reputations and some of humanity’s boldest scientific aspirations will be riding with it when it lifts off from French Guiana on Dec. 18 on an Ariane 5 rocket, about a decade later than planned.
“It’s been a very long journey,” says Smith, noting that his daughter, who was born shortly after he started working on the concept 25 years ago, recently received her graduate degree.
The first days after Webb’s launch will be tense for everyone who works on the project.
Once aloft, set up and commissioning will take about six months, and then Webb will begin attacking its top scientific goal of seeing 99.3% of the way back to the Big Bang. Also, its infrared-sensing instruments and large light-collecting mirror are ideal for observing exoplanets and specifically for measuring the compositions of these alien atmospheres. And astronomers will wield Webb to peer inside dusty stellar nurseries to gather new details about star and planet formation, as well as study the moons of the outer planets in our solar system and the faint objects way out in the Kuiper Belt, home to Pluto.
Delving deeper
To date, the most distant object humankind has ever managed to glimpse is the galaxy GN-z11 discovered in 2016 by astronomers poring over Hubble’s infrared observations. The observations show GN-z11 as it existed nearly 97% of the way to the Big Bang. This galaxy is a strange beast. It holds only about 1% of the mass of our Milky Way galaxy but furiously forms stars 20 times faster. While the extreme star formation rate fits with theoretical expectations for early galaxies, GN-z11 is unexpectedly large for its era, suggesting that key, unseen, galaxy-building steps preceded its emergence.
Hubble captured just enough light emitted by this galaxy’s stars to perform spectroscopy and obtain a redshift reading, which reveals a cosmic object’s distance. The spectroscopic process begins with the ridges on a glass surface, called a grating. This device divides the incoming light into spectra and directs the spectra to detectors that convert the photons into electrical signals. The color-coded lines that represent the spectra on an astronomer’s computer show a predictable pattern, usually from chemical elements emitting and absorbing particular wavelengths of light. In the special case of extremely distant and faint objects like GN-z11, astronomers see a specific transition in the spectral signature of hydrogen. This pattern shifts toward the infrared the farther away an object is, because the expansion of the universe has stretched the light out as it traveled to us. As a result, what had originally been emitted as ultraviolet light can redshift into longer (redder) visible wavelengths and, for highly distant objects, all the way on into the infrared, leaving no signal at all in the wavelengths of light that the signal originated. In the case of GN-z11, its degree of redshift shows that Hubble saw the galaxy as it appeared 13.4 billion years ago, or just 400 million years after the cosmos’ origin in the Big Bang.
As faint as it is, GN-z11 is nevertheless likely to be an ultrabright outlier for its era; in general, the farthest Hubble and the best ground-based observatories can strain to see back to is about 500 million years post-Big Bang. With Webb, however, astronomers expect to routinely see objects farther away than GN-z11, and possibly even pierce all the way to a mere 100 million years after the cosmos’ genesis.
Those few hundreds of millions of years matter tremendously, Smith points out. Galaxies are theorized to have grown in ways akin to human maturation, starting out small and then enlarging and developing more complexity in terms of their structure. Smith compares the galaxies that Hubble can just barely glimpse to “teenager galaxies,” while Webb will snap childhood pics, perhaps even baby pictures, unveiling a fledgling galactic era that’s been hidden to astronomers.
“When you think of babies, it’s an important time in a human life, where a lot of change is happening,” Smith says. “We think the same is true with galaxies.”
In keeping with the deep-field approach, Webb will delve deeper into some of the very same fields plumbed by Hubble in search of even more distant, younger galaxies. The project is called JADES for the JWST Advanced Deep Extragalactic Survey. Astronomers in charge of Webb’s three main instruments will coordinate with each other to systematically perform deep imaging and spectrometry on thousands of galaxies.
Those three instruments are the Near Infrared Camera, or NIRCam; the Near Infrared Spectrograph, or NIRSpec; and the Mid-Infrared Instrument, or MIRI. Together, the instruments cover a wavelength range of 600 nanometers (millionths of a meter) to 28,500 nanometers — spanning the orange portion of the visible spectrum out to the mid-infrared.
To trace early stellar and galactic growth, Webb will see light that was emitted as ultraviolet and visible light, but whose wavelengths have been stretched out into those ranges targeted by Webb’s instruments.
Because massive, newborn stars are particularly bright in the ultraviolet and visible ranges, the infrared light detected by Webb will serve as a marker of new star birth and as a tracer of galactic structure. The universe is expected to have undergone an initial surge of stellar birth as the matter formed in the Big Bang at last cooled enough to pool into clumps that then gravitationally collapsed into the nuclear fusion factories that are stars. Webb could see vast collections of stars — perhaps collectively even the first stars, formed almost purely of hydrogen — turning on together as the first galaxies.
Cooking up galaxies
As for galaxies themselves, they are more than just mere collections of billions of hosted stars. Most of a galaxy’s constituent material is dark matter, a poorly understood substance whose existence is inferable by the gravity it evidently exerts. All the observable expected matter, plus unobservable expected matter, in a galaxy amounts to a fraction of the cumulative gravitational force needed to keep a galaxy from flying apart; dark matter fills that yawning gap, outnumbering normal matter 6:1. Also playing a major role in galactic evolution are supermassive black holes. Almost every galaxy is thought to have one of these behemoths in its core, and the energy poured out of the region surrounding a black hole as the object devours matter can disrupt star formation throughout an entire host galaxy.
How and when regular matter, dark matter and black holes all came together as proto-galaxies and evolved into the common, spiral and football-shaped varieties of galaxies we see in the nearer, modern universe is a story whose beginning remains obscured. A program dubbed COSMOS-Webb, which has been awarded the most planned observation time, will help resolve the issue.
The program will take in a huge and representative sample of ultra-distant galaxies across a large swath of sky covering the equivalent of three full moons. This approach is very different from the extreme drilldowns of deep fields, which zero in on a spot “covering the same area of sky as that covered by the head of a pin held at arm’s length,” says Caitlin Casey, an astronomer at the University of Texas at Austin and the principal investigator for COSMOS-Webb.
Casey says Webb’s broader view of the distribution of a few tens of thousands of galaxies will reveal critical details about how the universe took shape.
“We’ll see if the galaxies forming shortly after the Big Bang live in the equivalent of cosmic metropolises, with lots of galaxies turning on together, or are galaxies roughly evenly distributed like in small hamlets across the universe,” says Casey. “Whether things are highly concentrated or not makes a big difference in our understanding, not just of how galaxies form and evolve, but how the universe came to be in those early days after the Big Bang.”
The search for life
As part of its science agenda, Webb will also stare intently at objects formed much later in cosmic chronology: nearby exoplanets. That Webb will be so useful in this regard is serendipitous, as exoplanetary ambitions had not factored into its design. NASA’s Smith jokes that in the mid-1990s, “when Webb was conceived, the universe only had two exoplanets.” Since that time, the field of exoplanetology has exploded, with at least 4,400 confirmed worlds known so far and many thousands of candidates awaiting additional verification.
Within this vast catalog of worlds, astronomers will train Webb on a select group of exoplanets around nearby stars. Some of those worlds have bulk properties of mass and size, coupled with temperate orbital distances from their host stars, that collectively indicate the planets could be habitable, even Earth-like. Knowing what these appealingly small worlds are really like is beyond the capabilities of current instruments, which cannot scan atmospheres for so-called biosignatures — mixtures of gases that could plausibly exist only due to biological activity.
Webb, though, with its infrared vision and large light-collecting capabilities, should prove ideal for transmission spectroscopy. This technique involves observing exoplanets as they cross the faces of their stars. During these transits, starlight filters through the planet’s atmosphere en route to Webb. Chemicals in the atmosphere absorb some of the starlight, leaving telltale spectral signatures.
Researchers hope Webb serves as the breakthrough tool for strongly inferring the existence of alien life. “The infrared is a good place to look for certain chemicals, like water, carbon dioxide, and methane,” says Smith. “Sometime in the not-too-distant future, because of Webb, you might be able to go out and look up at a star in the sky and say there’s a planet over there that could be habitable.”
Surmounting the technical challenges
None of this groundbreaking science would be possible without engineers having solved the immense problems posed by a large, infrared-optimized space telescope. To achieve their science objectives, planners settled on Webb having a 6.5-meter mirror, or about 2.5 times the diameter of Hubble’s 2.4-meter mirror, ultimately bestowing Webb with about six times its predecessor’s collecting area. To collect extremely faint, cold infrared light, the infrared-detecting portion of the space-borne facility must be cooled to temperatures on par with the surface of Pluto. To go so low, Webb’s mirror and instruments must be shielded from light from the sun, Earth and moon. That will be accomplished with a sunshield, measuring 21 by 14 meters (70 by 47 feet), or about the size of a tennis court, that keeps the infrared-detector side of Webb in shadow.
Given that the largest available rocket fairing was that of the Ariane 5, with a 4.57-meter internal diameter, engineers faced the unenviable task of designing Webb such that it folds up, Transformer-like, to be stowed for launch and subsequent unfolding in space.
“It was this combination of size and cryogenic operation of the telescope that conspired to make [Webb] a monumental engineering challenge,” says Paul Geithner, a deputy project manager at NASA for Webb who has worked on the project since 1997.
The mirror accomplishes its downsizing courtesy of two collapsible wing-like side sections. Meanwhile, the sunshield must pack perfectly, folding onto itself rather like a parachute. All these parts and pieces must then unfold and interlock with precision for Webb to see objects at unprecedented distances and as they existed billions of years ago.
“We’re essentially rebuilding and realigning the telescope on orbit,” says NASA’s Mike Menzel, Webb’s mission systems engineer, whose project tenure goes back to 1998 when he was an engineer at Lockheed Martin during the company’s early work on Webb; Menzel then joined Northrop Grumman, Webb’s prime contractor, in 2001 before coming to NASA in 2004.
The sunshield proved one of the highest hurdles. Engineers fashioned it as five layers of Kapton, a thermally stable polymer film, with each separated from the other by the vacuum of space. This architecture makes each layer successively cooler than the last by radiating absorbed heat back out into space. The sunshield works so effectively that it reduces a near-boiling temperature of 85 degrees Celsius (185 degrees Fahrenheit) from sunlight at its top layer to minus 233 degrees Celsius (minus 388 degrees Fahrenheit) on its cold, science operations side. To stow the sunshield, engineers placed a total of 107 pins through holes in the five layers; once in space, the pins release, and 90 tensioning cables throughout the sunshield move the heat barrier into its intended shape.
As for the primary mirror, engineers opted for beryllium because of its light weight, sturdiness and thermal stability. The mirror is divided into 18 hexagonal segments, each measuring 1.3 meters across, arranged in a honeycomb-like pattern, and coated with a highly reflective, microscopically thin layer of gold. The mirror has two foldable wings consisting of three vertically aligned segments each. When folded away from the 12-segment core, the wings shave off about 2.6 meters off the mirror’s diameter to fit in the Ariane 5. Each mirror segment has seven motors, or actuators, to precisely control the segments’ alignment, plus an additional actuator that can fine-tune the curvature of each segment, all working in concert to focus the telescope.
Another design tactic to reduce the temperature on the science side was to place Webb’s heat-generating main spacecraft bus, with its solar panel, communications antenna, computer, and reaction wheels and jets all on the hot side of the sunshield.
Compounding the challenge of fashioning all this hardware was having to build Webb’s components here on Earth in ambient room temperatures, though portions of the spacecraft operate in deep cryo. Accordingly, engineers had to account for how every material composing Webb would change shape as the mercury plunges. Doing so meant building in tolerances, along with small heaters to allow for a mission controller-guided cooldown.
“We had to build Webb perfectly wrong at room temperature so it’s perfectly right at its operating temperature,” says Geithner.
To additionally assist in keeping Webb cold, mission planners chose to place Webb in an orbit about the second Lagrange point, or L2, a waypoint 1.5 million kilometers outward from Earth where the gravitational infuences of our planet and the sun balance the orbital motion of an object. There, Webb will face away from the sun while expending little energy on course corrections.
During the first two weeks in space, a series of complex deployments will take place as Webb makes the monthlong journey to L2. Once there, the actuators will bring the segments into alignment and to their correct curvature, followed by a commissioning phase. Mission planners have taken to calling this tense post-launch period the “six months of terror,” an allusion to the well-known seven minutes of terror experienced by Martian probes undergoing entry, descent and landing. Unlike the completely autonomous EDL at Mars, though, necessitated by the communications time delay with Earth, Webb is “never more than a few light-seconds away,” says Geithner, meaning mission controllers will be directly implementing and overseeing every phase of the commissioning process. “We have a huge degree of flexibility in executing our plan, depending on if something weird happens,” says Geithner. “But we’re hoping it’s really boring.”
The long wait is over
For all the scientists and engineers who have played a role in realizing Webb for a quarter-century now — equal to the bulk of the careers of Smith, Geithner and Menzel — the imminent launch is tantalizing. “It’s surreal to be this close to actually doing what we set out to do,” says Geithner.
If the science returns can meet expectations, many in the astronomical community might justify Webb’s $8.8 billion development price tag and well over a decade of delays. “The wait has been worth it,” says Casey.
Smith admits to mixed feelings, having spent so long connected to the observatory here on the ground. Yet he knows the best part, the science, will start soon.
“Webb feels like one of your children when they go off to college,” Smith says. “Some part of you hates to see them go, but part of you knows it’s where they belong.
“I’m going to miss the observatory. It’s bittersweet,” adds Smith. “But Webb wants to be in space. I can’t wait to see what this amazing instrument can do.”
Related Topics
Space ScienceThe price of Webb
Development and construction totaled $8.8 billion, according to NASA, but a space telescope on the ground is of course of no use, so launch and operations must also be considered. The European Space Agency early on agreed to provide an Ariane 5 launch vehicle at no cost to NASA. As for operations, NASA plans to spend $900 million operating the telescope for at least five years, which brings the mission cost to $9.7 billion.
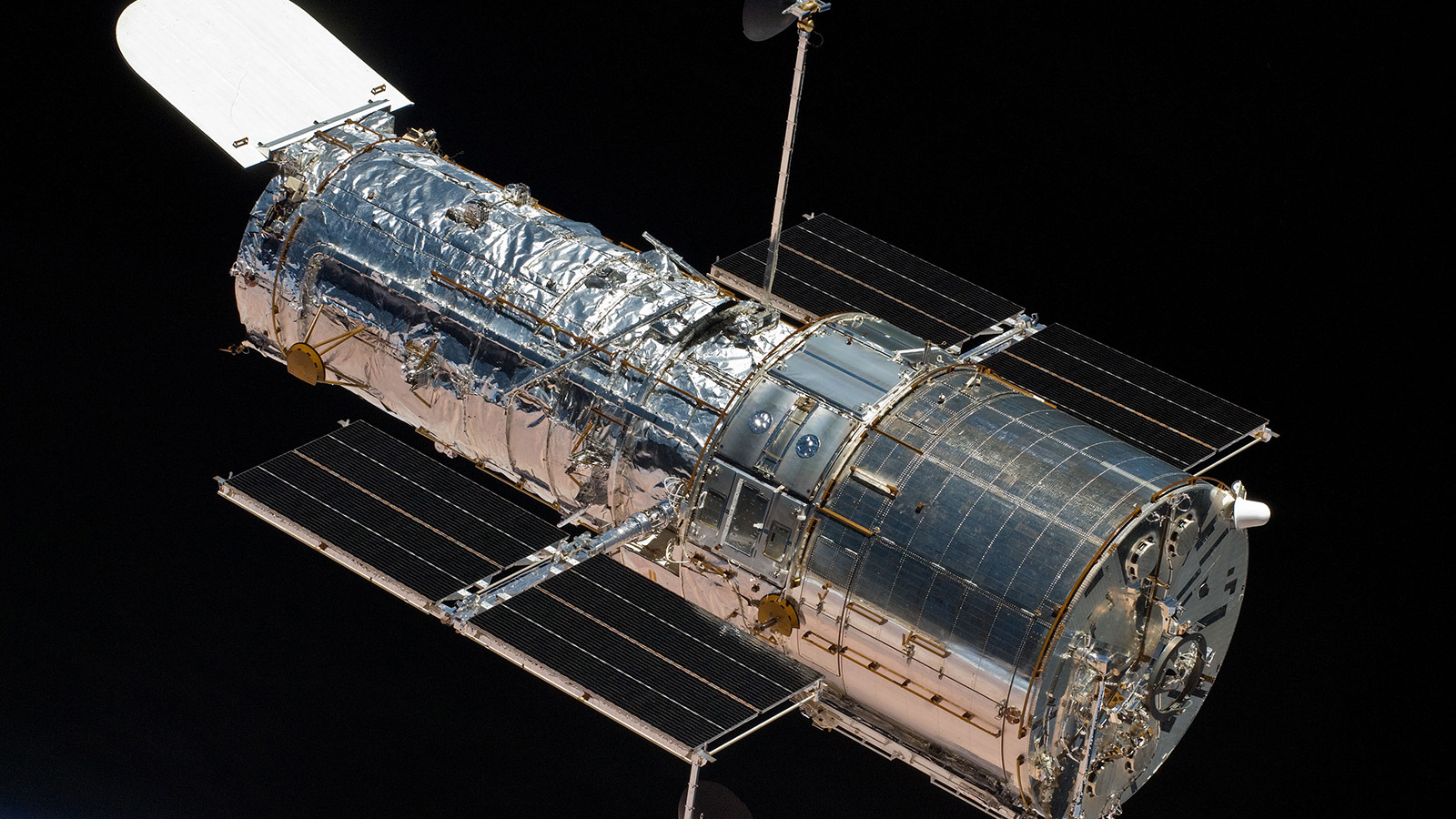

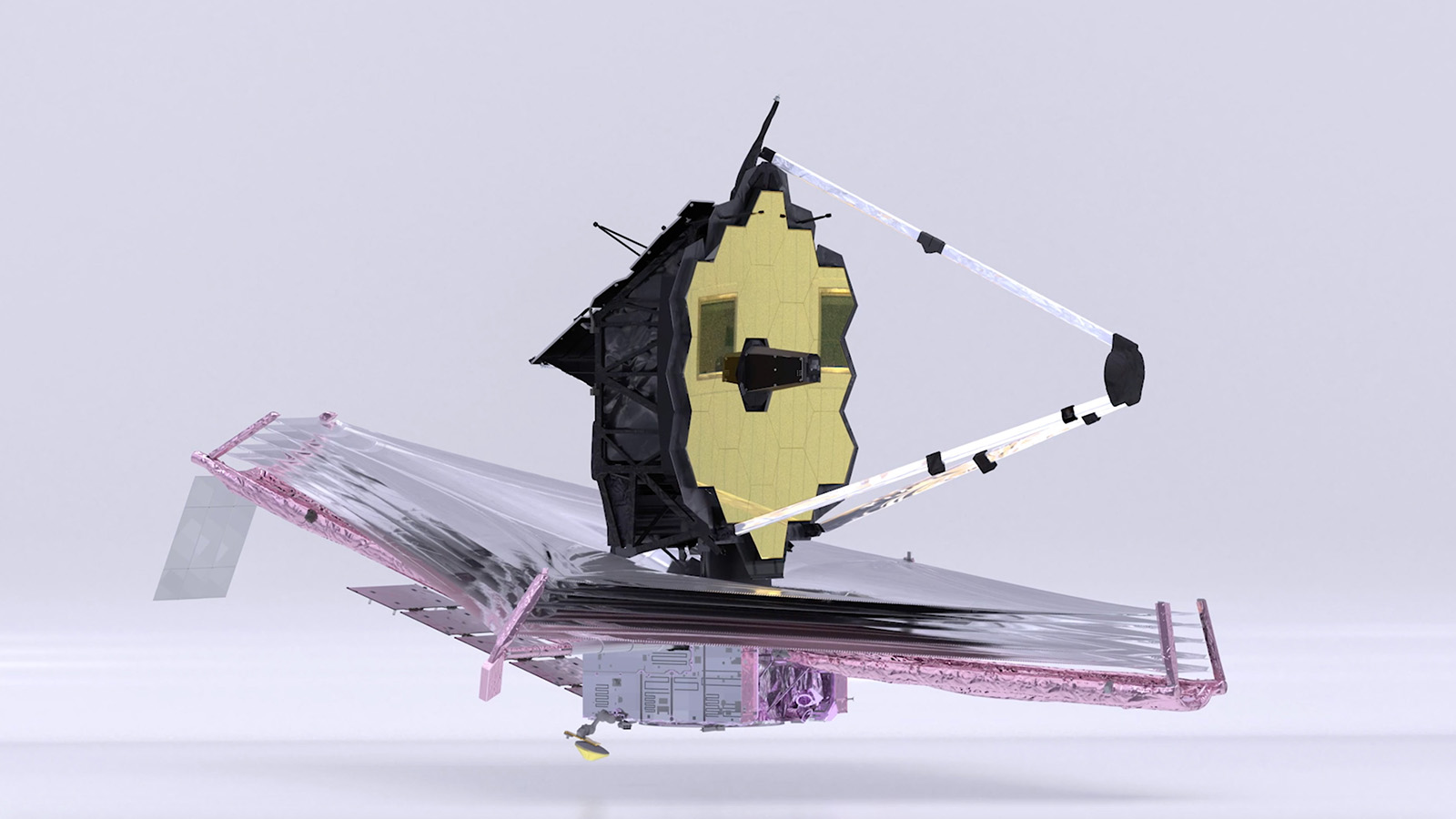