Designing Dragonfly, NASA’s Titan explorer
By Paul Marks|October 2022
A planetary lander that can fly to new sampling sites will be able to do far more surface science than a slow, trundling rover. Easy flight in the super-dense atmosphere and ultra-low gravity of Saturn’s cryogenic moon, Titan, makes it the perfect celestial body on which to test the idea. Paul Marks spoke to the team behind NASA’s Dragonfly.
In 2034, high above Saturn’s largest moon, the handiwork performed years earlier by engineers at a laboratory in Maryland will snap into action, if all goes as planned. After a six-and-a-half-year cruise through deep space, punctuated by a gravity assist from Venus, a NASA-funded probe will plunge into the thick orange haze of Titan’s upper atmosphere, a heat shield protecting the planetary explorer ensconced in its aeroshell from the incandescence of atmospheric entry.
This explorer, Dragonfly, will be unique in the annals of space exploration. An octocopter roughly the size of a Mini Cooper, it will fly out of the aeroshell and descend to its nominal flight altitude of 400 meters, where it will train lidar and high-resolution cameras on the terrain below to seek a safe, solid landing spot.
Dragonfly’s descent won’t be anything like the complex “seven minutes of terror” skycrane deployment NASA’s Curiosity and Perseverance rovers had to endure to land on Mars.
“Our entry, descent and landing profile on Titan is more like two hours of concern,” says Ken Hibbard, mission systems engineer and technical lead for Dragonfly at the Johns Hopkins University Applied Physics Laboratory in Maryland.
Why so long? Titan’s smog-laden atmosphere extends up to an altitude of over 1,200 kilometers, or 10 times higher than the atmosphere of Mars. At the surface, its density is 4.3 times that of Earth’s, and its atmospheric pressure is 1.4 times higher. And with only one-seventh of Earth’s gravity gently tugging the spacecraft down, the consequence is a very slow descent indeed.
Once safely on the surface, Dragonfly can begin what its designers hope will be more than three years of science operations at dozens of sites. After landing, the drone will sample the surface material beneath it, analyze its chemistry and beam its data to Earth. But unlike wheeled rovers, it can then start its rotors again and fly off to some very different, and very distant, geological locations — using its cameras to navigate optically, informed by mapping data gleaned from flybys of NASA’s Cassini probe — and do another batch of surface science.
It’s a three-year mission because one day on Titan, known as a T-sol, is 16 days on Earth — and Dragonfly’s nominal mission length is slated at 74 T-sols, which equates to 3.2 terrestrial years. And in that time, says Hibbard, it could have flown at least 180 kilometers, perhaps more. That’s an order of magnitude more than Curiosity, which has managed 28 kilometers over its decade on Mars.
And it is this science return that has dictated much of Dragonfly’s engineering design. So what will scientists be trying to learn from it? The answer: a whole lot about the primordial soup that led to the emergence of life on Earth.
“Titan might be the equivalent of the prebiological chemical state of Earth billions of years ago,” says Hibbard.
A shade bigger than Mercury, Titan is deemed the most Earth-like celestial body in our solar system because its dense nitrogen-based atmosphere — laden with methane and a complex hydrocarbon haze of ethane, propane, ethylene and methacetylene — supports a system that’s in some ways comparable to Earth’s hydrological cycle. But it is based on the condensation of methane, rather than water: On Titan, clouds, rain, rivers, lakes and seas run with liquid methane, thanks to the moon’s cryogenic surface temperatures of minus 180 degrees Celsius, mainly a result of it being more than nine times farther from the sun than Earth is, and global cooling due to the haze.
And there is evidence from Titan’s crater morphology that asteroid and comet impacts may have melted water ice. That liquid water could therefore have persisted in the resulting craters for extended periods — perhaps for tens of thousands of years — due to the impact heat, says Dragonfly principal investigator Elizabeth “Zibi” Turtle of the Applied Physics Lab, or APL.
And that adds up to some very tantalizing possibilities. For one, “Titan has on its surface, or has had in its past on its surface, all of the ingredients we know to be necessary for life,” says Turtle.
With sunlight driving complex chemistry among large hydrocarbon molecules in Titan’s upper atmosphere, some of the products of those reactions fall to the surface, which is riven with wind-sculpted dunes comprised of mysterious organic, sandy particulates of unknown makeup. APL has not been able to create these grainy substances in the lab. And if they meet water from Titan’s subsurface ocean or impact crater meltwater, “you’ve got liquid water and organic material mixing for extended periods of time, much longer than we can do experiments in the laboratory,” says Turtle.
“And so Titan has been doing experiments in prebiotic chemistry — the chemical steps that enabled chemistry to take the leap to biology — possibly over millions of years. And really what Dragonfly is designed to do is to go pick up the results of those experiments.”
Designing a relocatable lander
To date, the only craft that’s touched down on Titan’s surface is the European-built Huygens lander in 2005 that hitched a ride aboard NASA’s Cassini probe, but that spacecraft was not designed for longevity and its batteries died a little over an hour after its parachute-assisted descent. The goal of engineering another mission to Titan has sparked some ambitious ideas over the years, Hibbard and Turtle say, including a boat or submarine that could travel in its cryogenic oceans or balloons, helicopters and airplanes that could fly to multiple locations in the dense, lift-generating atmosphere.
The APL team preferred a mobile explorer because Cassini, having mapped Titan over the span of 13 years and 126 flybys, found the terrain to be too highly varied to gain a broad enough spread of science results at a single rover site. There are, for instance, widely separated regions of organic sand dunes, broad and flat interdune spaces, methane and ethane rivers, lakes, seas and numerous impact craters. How could such a craft be controlled stably and also autonomously fly the many hundreds of kilometers between these sites? And how could it be powered, given that Titan’s haze makes the sun 100 to 1,000 times dimmer than on Earth?
In 2016, when NASA said it would consider Titan missions under its New Frontiers program, some members of the APL team realized that the convergence of two aerospace technologies suddenly made a rotorcraft an option for Titan.
“The drone revolution had happened, and so an autonomous rotorcraft was no longer a crazy idea,” says Ralph Lorenz, a planetary scientist at APL. He recalls hatching the initial idea for a multirotor Dragonfly in a conversation with his colleague Jason Barnes, a planetary scientist at the University of Idaho. The pair hashed out how such a craft might work over a group dinner at a Texas restaurant after a NASA Outer Planets Assessment Group meeting.
Another fortuitous development: A new kind of plutonium power source, the Multi-Mission Radioisotope Thermoelectric Generator, had been developed by Aerojet Rocketdyne and Teledyne Energy Systems under a U.S. Department of Energy contract and was being made available to NASA missions that needed one. One MMRTG had already flown on Curiosity, and another was planned for the (then upcoming) Perseverance rover.
“I had a head-slap moment, namely the realization that a rotorcraft of some sort would be a perfect fit with the MMRTG that NASA would make available,” Lorenz says. “I had advocated Titan helicopters 16 years earlier, before the MMRTG had been developed, but the idea had seemed fanciful, and there wasn’t then available a power source that would work in a planetary atmosphere.”
In 2019, NASA gave the Dragonfly project the go-ahead and a budget of $850 million, excluding launch and operations costs. Lorenz is now mission architect for Dragonfly, and Barnes is the program’s deputy principal investigator.
Currently slated for a launch in June 2027 and arrival at Titan in 2034, Dragonfly is the second NASA-commissioned planetary exploration rotorcraft after the Ingenuity helicopter, which landed on Mars slung beneath Perseverance in 2021. During the 32 flights Ingenuity has made as of September, it has demonstrated the sheer usefulness of dispatching a camera-equipped air scout to assess terrain for potential sampling. And underscoring this success, in a change to NASA’s Mars Sample Return program, mission planners announced in July they’ve decided to send two new uprated “Ingenuity-class” helicopters aboard MSR’s Sample Retrieval Lander, scheduled to launch toward Mars in 2028. Unlike the first Ingenuity, each would be equipped with wheels so they could traverse the surface and robot arms so they could grasp and carry sample test tubes — basically assisting Perseverance when needed.
And there is fruitful cooperation between the rotorcraft teams at APL and NASA’s Jet Propulsion Laboratory, where Ingenuity was built and tested: “As we’ve been developing Dragonfly, we’ve had a lot of really good, healthy interactions with the Ingenuity team,” Hibbard says. “They’ve been very supportive of us, and we’ve learned lessons from them. And some of their chief engineers are on our review boards.”
Indeed, Dragonfly’s major subsystems faced preliminary design review analyses in July and August. So what does Dragonfly currently look like?
Dragonfly [See diagram] comprises a lander body supported on skids, measuring 2.4 meters high from the ground to the top of a deployable high-gain antenna used for communicating with Earth. Four outriggers, each carrying two motors and two rotors, project from each side of the lander body, making the 450-kilogram octocopter 3.7 meters wide. And from the nose to the MMRTG containment capsule at the rear, it also measures 3.7 meters.
One of the issues under the microscope is how, in Titan’s excruciatingly cold ambient temperatures of minus 180 Celsius, Dragonfly’s electronics inside that lander body can be kept anywhere near warm enough to function well. On Mars, by comparison, Ingenuity basks in a toasty minus 60 C.
An early problem the Dragonfly engineering team overcame was how to customize its MMRTG power source. As supplied, the unit normally comprises a cylinder containing a radioactive heat source and thermocouples that use a semiconductor junction to convert heat into a current, generating 110 watts of electricity. Fins the length of the cylinder usually radiate away waste heat.
On ultra-frigid Titan, however, heat is valuable.
“Because the MMRTG has about 2,000 watts of thermal power that are waste heat, we actually try to trap all that inside our lander to keep it warm,” Hibbard says. “So we’ve cut the fin length in half because we don’t want to radiate that heat away. And we have a series of fans that pull the waste heat from the MMRTG and distribute it under the lander body up to the nose.”
What’s vital with the thermal management of Dragonfly is to ensure that the science platform at the heart of the machine does not freeze up. That science package comprises:
- a mass spectrometer to identify every chemical in samples vacuumed up from the surface,
- a neutron-activated gamma-ray spectrometer to assess the broad elemental composition of the surface Dragonfly is sitting on,
- a seismometer to measure Titan’s quake activity and subsurface structures,
- meteorological and atmospheric sensors, and
- 10 cameras and two lidars to image geological features and provide imagery and altitude and ranging measurements for navigation.
Insulating all these instruments is an ongoing issue. “We’re still trading some of the material choices, as we’re in preliminary design,” says Hibbard.
Traditional spacecraft materials such as titanium, aluminum, aluminum honeycomb and magnesium are in the frame for the structures. What looks certain is that the entire lander body is to be coated in a 7-centimeter-thick layer of an aerospace-grade insulating plastic foam, made from polymethacrylimide and known commercially as Rohacell, which gives renderings of the drone their distinctive coppery, tan color.
The aim of all the ongoing thermal design trades, says Hibbard, is to ensure Dragonfly’s interior remains in a range from minus 40 C to 30 C, and that its lithium-ion battery, in particular, is kept at its preferred temperature of around 20 C. “So most things inside will be somewhere between zero and 30 degrees Celsius,” he says.
And to ensure that the 11.5 kilowatt-hour battery pack is as reliable as possible — it will be trickle-charged by the MMRTG to power all Dragonfly’s systems — APL is building it using large, high-energy-density cells made by GS Yuasa of Japan, whose li-ion batteries were deemed reliable enough to have been fitted spaceside on the International Space Station. With a trusted human spaceflight technology like that on the market, “we didn’t want to invent new technology,” says Hibbard.
Less bother in the hover
Where APL does need to innovate, however, is on the propulsion side, though they are aided hugely by the munificence of Titan’s physics. Thanks to the moon’s high atmospheric density and low gravity (again, 4.3 times more dense than Earth’s and one-seventh the gravity), the power needed to make a rotorcraft of a given mass hover on Titan is, astonishingly, only 2.5% of the power it would take to make the same aircraft hover on Earth. “Which is why Titan is such an ideal place to fly,” says Hibbard.
So just how big should the rotors be? They would need to provide adequate power, but making the rotors longer than necessary would waste valuable mass and volume. So the engineers plugged in Titan’s gravitational acceleration and atmospheric density into their rotorcraft math, in which hovering power is inversely proportional to the square root of the total swept area of the rotor disks. The APL team, knowing the volume of the 5-meter-diameter aeroshell Dragonfly must fit in for its launch and cruise from Earth, could work out some options. They did so for a quadcopter and an octocopter, and it turned out that rotors 1.35 meters long would do the optimum job.
They also chose the octocopter option. “Redundancy, more than anything, really drove us,” says Hibbard. “With the octocopter, we can lose one rotor and still fly.”
One reason Dragonfly might lose a rotor (or a motor) is the mysterious organic sand that the APL team suspects Titan’s dunes are made of: If it’s a hard particulate, debris kicked up by the rotors on multiple landing and takeoff cycles could eventually damage the rotors, especially if embrittled by the cryogenic temperatures. So one option under consideration is to make the rotors from aluminum but with a tough titanium leading edge, says Hibbard. That said, the team is also keeping an eye on NASA research into cryogenic-temperature-tolerant composites — a welcome weight saving compared to a composite rotor.
The brushless DC motors that will drive those rotors are being custom made by Moog Inc. of New York. They are being dual wound, for redundancy, and are “being designed specifically for Dragonfly to provide the necessary torque-to-mass ratio for our blades,” says Hibbard.
But when Dragonfly lands to do surface science, its electric motors, stuck out in Titan’s atmosphere on those outriggers, will need to be able to survive the cryogenic temperatures when they are switched off. To cope with this, Hibbard says the motors will contain a specially designed lubricant that can freeze without causing internal damage. When it’s flight time, mission controllers simply activate heaters to warm the motor up to operational temperature so it can fly to a new science site.
When it gets there, a novel vacuum-based sampling system gets to work. Instead of the robot arms on a rover like Perseverance, Dragonfly will have rotary percussive drills to break up surface material on either side of its front skid support, alongside tubes to suck up the debris.
“Because we have this great atmosphere on Titan, we can use pneumatic transport,” says Turtle. “We just vacuum up the material into the mass spectrometer.”
But how do the engineers ensure that materials sucked up from one site do not contaminate samples from other sites that Dragonfly flies to? After all, when seeking prebiotic chemicals — precursors to amino acids and perhaps proteins — knowing where they were found will be vital.
The answer lies in some smart single-use technology in the mass spectrometer itself: “The pneumatic sample transfer system uses one-shot [sample] cups to minimize the site-to-site crosstalk,” says Lorenz. “We can also run the blowers that suck the air through the system for an extended period before and after a sampling operation to flush any material out of the pipes.”
Alongside the preliminary design review of every Dragonfly subsystem and instrument — including the sampling system — APL has also begun test flights with a half-scale octocopter in the physically (if not thermally and atmospherically) similar landscape of California’s Imperial Dunes, west of Yuma, Arizona.
“The scale model tests at Yuma are only for exercising the optical navigation system on representative terrain,” says Lorenz. “What have we learned? That the system works well. A complication in the tests that we won’t have on Titan where the illumination is very diffuse, is that the system can lock onto the lander shadow as a false ‘fixed’ feature in the navigation images.”
It’s clear from talking to the Dragonfly engineers that they are a passionate, imaginative team. So with those wheels and robot arms being added to the new Ingenuity-class helicopters for Mars Sample Return, is there any temptation to let their imaginations fly and make late additions to Dragonfly’s capabilities?
Apparently not: Dragonfly’s design includes everything its science objectives demand and its New Frontiers budget can cope with, says Turtle.
“So at this point, we are not looking to make additions to the payload. Although we can all of course think of other interesting experiments to do, those will lead the way for future missions.”
Related Topics
Space Science“Titan has on its surface, or has had in its past on its surface, all of the ingredients we know to be necessary for life.”
Elizabeth "Zibi" Turtle, Johns Hopkins Applied Physics Laboratory
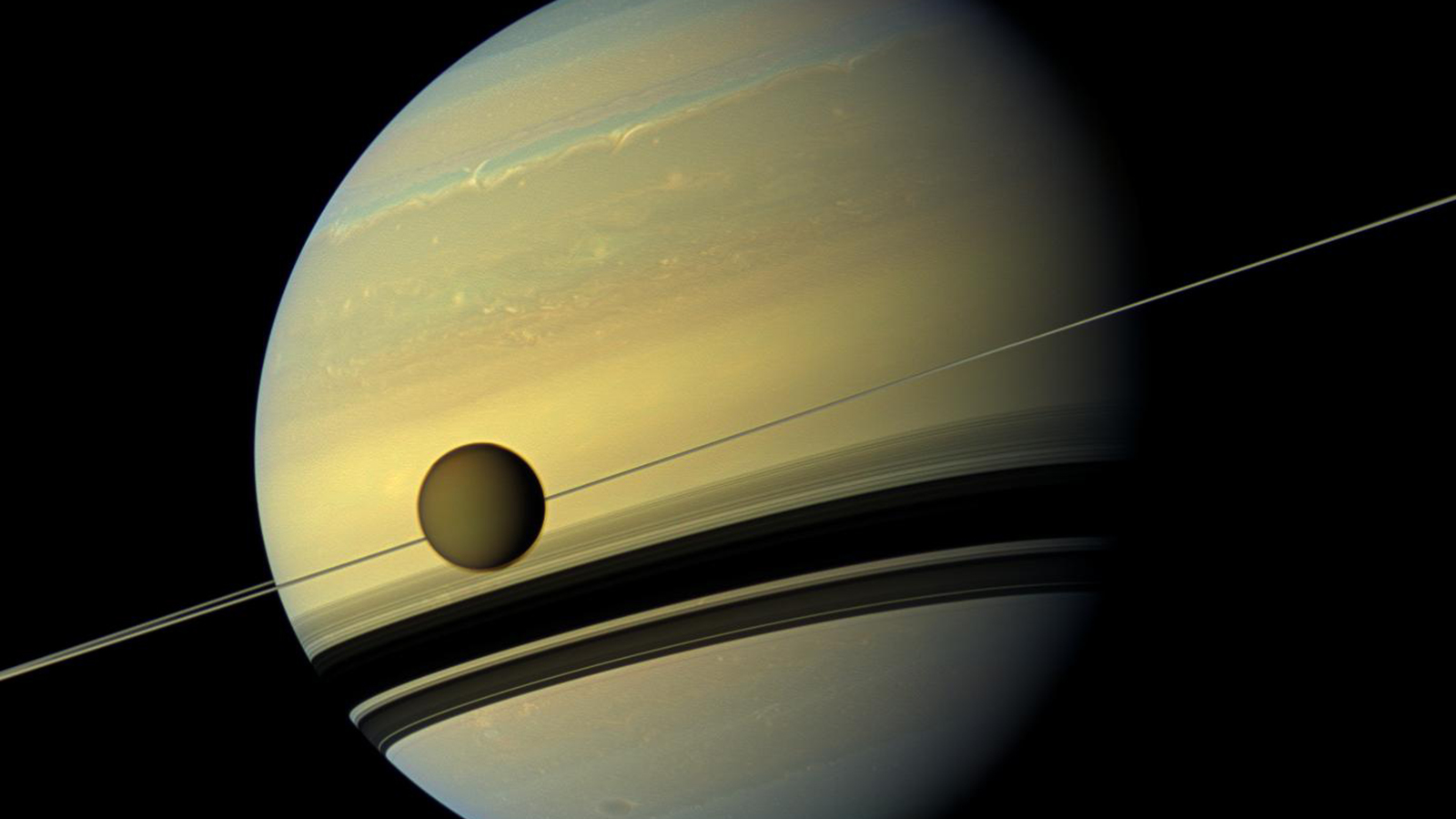