Dancing cubesats
By Debra Werner|July/August 2017
Coming up with best means of squeezing propulsion into a tiny package
Space-industry watchers have little doubt that the tally of cubesat launches in 2017 will demolish the previous record set in 2014 when 132 of these miniature satellites were launched. Already, government agencies, universities and companies have launched 142 cubesats as of June 1.
Once seen mainly as a way to give students hands-on experience, cubesats have proved so useful that NASA, the U.S. Air Force, intelligence agencies and Silicon Valley startups are devising multimillion-dollar Earth imaging and communications missions around them. With that newfound importance, come challenges. When cubesats were humble teaching tools, professors were satisfied with whatever data they could obtain before their cubesats succumbed to drag and burned up in the atmosphere. With millions of dollars and key objectives now on the line, many cubesat developers want their spacecraft to have propulsion.
Cubesats could then maneuver to their optimal orbits instead of making the best of their drop-off points after hitching a ride on a rocket with a much larger satellite. The cubesats could resist atmospheric drag and remain in space for years, and at the end of their missions, be de-orbited to avoid creating debris.
Designers would have new options. Constellations or swarms of maneuvering cubesats might take on many responsibilities traditionally reserved for bus-size satellites. Those constellations could be established rapidly. Today, it takes months for San Francisco startup Planet to move its newest cubesats into position through differential drag. With propulsion, that would take just days. For missions beyond Earth orbit, cubesats destined for asteroids, the moon or Mars already are being designed with onboard propulsion.
“Cubesats are doing the same kind of jobs the big satellites are doing. So in the same way that propulsion is good for the big guys, it’s good for the little ones,” says Jordi Puig-Suari of the California Polytechnic State University, who in 1999 developed the cubesat standard together with Bob Twiggs of Stanford.
The task now is to figure out which new thruster designs will work. “We are dealing with rocket science and plasma physics,” says Natalya Bailey, founder and chief executive of Accion Systems, a Boston startup focused on electric propulsion for small satellites. “People can put forth spec sheets and claim things, but flying the engines is going to be the main differentiator.”
Some concepts are forms of solar electric propulsion, in which solar cells on the exterior of the cubesat would convert sunlight to electricity that can be applied in a variety of ways to create propulsion. Other concepts are takeoffs on traditional chemical propulsion. Here’s a look at some of the technologies on the horizon:
Hall-effect thrusters
These thrusters are named for American scientist Edwin Hall who described how magnetic fields can shape electrical currents. Hall-effect thrusters trap electrons and make them collide with the atoms of a propellant, and the resulting ionized gas is accelerated with magnetic and electrostatic fields. Busek of Massachusetts, a company well-known for Hall-effect thrusters, is preparing to test one later this year on a 12U cubesat called Iodine Satellite or iSat for short. The thruster, developed with funds from NASA and the U.S. Air Force, will excite an iodine propellant with radio frequency electromagnetic fields. Cubesat developers, including those at the U.S. Air Force Space and Missile Systems Center in California, are eager to test iodine because it’s three times as fuel efficient as the commonly flown xenon. Iodine-fueled missions would pack the same power with one-third the fuel. Plus, iodine can be stored as an inert solid in low-pressure plastic tanks, while xenon requires pressurized metal tanks and pressure regulators.
ExoTerra Corp., a small business in Colorado established by some former Lockheed Martin engineers, has developed what it calls the Halo Hall Effect Thruster. Each would take up one-quarter of a 1U cubesat, weigh 0.48 kilograms, and could be fueled by either xenon or iodine.
“It’s the smallest Hall thruster we are aware of on the market,” says Michael VanWoerkom, ExoTerra founder and president. “We can package a kilometer per second of delta-V” — change of velocity — “into a 6U cubesat.”
In March, NASA awarded ExoTerra a $2.5 million contract to move forward on the technology under the agency’s Tipping Point Program, which aims to back projects on the verge of a breakthrough. NASA plans to flight test a 300 watt version of ExoTerra’s solar electric propulsion system on an asteroid-hunting cubesat scheduled for launch in 2019.
Electrospray thrusters
These apply a charge to an ionic liquid propellant before accelerating it with an electric field. Busek of Massachusetts developed the technology for a possible future NASA-European Space Agency effort to detect gravitational waves, the ripples in spacetime predicted in 1915 by Albert Einstein in his theory of general relativity. To make those gravity measurements, scientists will need a three-satellite observatory, and each of those satellites must be held in a precise orientation relative to each other and Earth. Even the most minute disturbances must be counteracted and that’s where Busek’s electrospray thrusters come in. NASA demonstrated this and other required technologies in 2015 on a satellite called LISA, short for Laser Interferometer Space Antenna.
Tyvak Nano-Satellite Systems, an Irvine, California, company founded by cubesat co-creator Jordi Puig-Suari, plans to incorporate a Busek electrospray thruster in a 6U NASA Pathfinder Technology Demonstrator scheduled for launch in 2018. The thruster measures 9 centimeters by 9 cm by 4 cm.
Accion Systems is developing a different kind of electrospray thruster based on technology its founders, Natalya Bailey and Louis Perna, developed at MIT’s Space Propulsion Laboratory. While earning doctorates, Bailey and Perna worked on prototypes of miniature ion engines that were flight tested on AeroCubes, cubesat technology demonstrators built by the federally funded Aerospace Corp. in Los Angeles, which conducts research and development for the U.S. Air Force. Soon, the U.S. Defense Department began funding MIT’s research, and aerospace prime contractors asked whether they could purchase flight systems or license the technology. That’s when Bailey and Perna decided to establish Accion. “We knew the trend was toward smaller and smaller satellites and we really felt it when people were knocking on MIT’s door trying to buy systems,” Bailey says. Since forming Accion in 2014, Bailey and Perna have raised $10.5 million from investors and obtained $6.5 million in funding from U.S. defense agencies, which the firm declines to name. The electrospray engines, which are roughly the size of a pack of cards, run on a salt-based liquid propellant that flows through hundreds of microscopic emitters housed in microchips that it prints in a manner similar to how computer chips are made. This manufacturing innovation wouldn’t have been necessary “if you needed to make one ion engine a year,” Bailey notes. “Given the trends in the industry, we thought it would be wise to pull mature manufacturing techniques from other industries.”
Ion thrusters
These accelerate ions with electrical power, and can be fueled by iodine or xenon. Busek of Massachusetts has created iodine-fueled ion thrusters that are scheduled to propel a pair of 6U cubesats to lunar orbit. One is called the Lunar Ice Cube cubesat and is built by Morehead State University in Kentucky. The other was built by Arizona State University and is called the Lunar Hydrogen Mapper. Both are slated to ride on the first flight of NASA’s Space Launch System rocket in early 2019. Once released, their thrusters will be so efficient that, if all goes as planned, they will easily reach lunar orbit even with the small amount of propellant that fits in a cubesat. In fact, they should have enough propellant left after reaching orbit to move closer to the moon for scientific observations, says Vlad Hruby, Busek president and founder.
Radio frequency thrusters
These convert electrical power into radio waves that act on a propellant to generate thrust. Phase Four, a 12-person startup near Los Angeles International Airport, has designed and is scheduled to test the Phase Four Radio Frequency Thruster. These thrusters convert DC into RF power, to ionize and heat xenon. The design does not need cathodes and anodes, relying instead on electronics similar to those in cellphones to generate radio frequency signals that heat and ionize the xenon into a plasma. Magnets then force this plasma through a nozzle to produce thrust. Soon after the company’s founding in 2015, Phase Four won a $1 million DARPA contract to build a prototype and associated components. The design is scheduled to make its orbital debut early next year on an agriculture monitoring satellite called Landmapper. It’s a 6U cubesat made by Astro Digital, a small company at the NASA Ames Research Park in California.
“From a business perspective, being able to say we have flight heritage will be a huge differentiator,” says Simon Halpern, the Phase Four founder.
In a sign of how anxious the industry is for cubesat propulsion, Phase Four has begun selling a plug and play model for cubesats even though the design has not yet been to space.
Resistojet propulsion
These concepts pass electricity through a resistor to generate heat that produces thrust differently depending on the design. Surrey Satellite Technologies Ltd. of Guildford, England, in 2013 flew its Water Alcohol Resistojet Propulsion, known as WARP Drive, on a cubesat called the Surrey Training Research and Nanosatellite Demonstrator 1. This was a joint effort with the University of Surrey’s Space Center. Heat produced by a resistor turns de-ionized water mixed with alcohol into superheated steam that is forced through a nozzle.
Micro-cavity discharge
Electrodes heat the propellant, turning it into plasma in a micrometer-diameter cavity before discharging the plasma through a nozzle. CU Aerospace of Illinois and VACCO Industries of California, have joined forces to create the Propulsion Unit for Cubesats or PUC for short. A PUC unit can be as small as one-quarter of 1U or 10 centimeters by 10 cm by 2.5 cm. A U.S. government customer that CU Aerospace and VACCO say they are not permitted to name purchased several PUCs four years ago for classified cubesat programs. “I was told that I will never know what happened,” says Cleve Samson, VACCO’s regional sales manager who specializes in the company’s micro-propulsion systems.
Chemical propulsion
Various groups are adapting the basic concept of emitting gases or vapors to generate thrust. VACCO Industries of California has developed a propulsion unit that releases R134a, a hydrofluorocarbon refrigerant, from a pressurized tank through eight thrusters, two at each corner of the module. The first two modules, enough to fill a 1U cubesat, are scheduled to fly for the first time in September on the NASA-funded Cubesat Proximity Operations Demonstration. If all goes as planned, two 3U cubesats will rendezvous, operate near one another and dock. The cubesats are made by Tyvak Nano-Satellite Systems of California.
VACCO has also delivered two propulsion units for the first cubesat built for an interplanetary journey, the NASA-funded Jet Propulsion Laboratory’s Mars Cube One, called MarCO for short. This 6U cubesat is scheduled to ride toward Mars with a lander called InSight, short for Interior Exploration using Seismic Investigations, Geodesy and Heat Transport. MarCO will stay in space to relay communications between the InSight lander and mission planners on Earth.
Cubesat developers can also buy miniature versions of propulsion systems that combine hydrazine with a catalyst to create thrust. Aerojet Rocketdyne, for example, drew on technology from its missile defense thrusters to build the Cubesat High-impulse Adaptable Modular Propulsion Systems, or CHAMPS, a thruster that fills a 1U cubesat and is designed for missions that need rapid acceleration.
“Hydrazine is very quick responding,” says Joseph Cassady, who directs Aerojet Rocketdyne’s space programs in Washington, D.C. “You can get a little pulse. That’s key for a lot of space missions because you are doing fine maneuvers to adjust the trajectory.”
With funding from NASA, Aerojet Rocketdyne qualified CHAMPS for flight and is looking for an in-flight demonstration.
Due to hydrazine’s toxicity to workers, some companies are experimenting with thrusters that will run on nontoxic “green” propellants. VACCO of California is developing a hybrid propulsion system for the Italian company Argotec’s ArgoMoon cubesat. VACCO pairs a green monopropellant warm gas thruster, fueled by Sweden’s Ecological Advanced Propulsion Systems’ High Performance Green Propulsion (which has an ammonium dinitramide base), to push the miniature spacecraft into its desired lunar orbit with four cold gas thrusters for attitude control.
Aerojet Rocketdyne’s green propulsion unit, fueled by a hydroxyl ammonium nitrate fuel/oxidizer blend, designated AF-M315E, is slated to fly in late 2017 on NASA’s Green Propellant Infusion Mission. To reduce costs, Aerojet Rocketdyne additively manufactured the unit’s structure and tank in one piece with mounting brackets for five thrusters. Instead of 50 to 75 parts, its monopropellant subsystem has fewer than 10 parts. “We had to find a way to get the price well below where our traditional propulsion systems would be for these guys to even consider buying it,” Cassady says.
The federally funded Aerospace Corp. in California has developed a nontoxic, nonflammable, nonexplosive, noncarcinogenic thruster fueled by water that is turned to steam. It is scheduled to fly on NASA’s Optical Communications and Sensor Demonstration, a two cubesat mission slated for launch later this year to test how well laser pulses can relay data between low Earth orbit and a telescope on top of Los Angeles’ Mount Wilson. “It’s hard to come up with a mode where a water thruster can cause a problem,” says Richard Welle, who heads the Aerospace Corp.’s Microsatellite Systems Department.
Related Topics
Spacecraft Propulsion“Cubesats are doing the same kind of jobs the big satellites are doing. So in the same way that propulsion is good for the big guys, it’s good for the little ones.”
Jordi Puig-Suari, co-creator of the cubesat standard
Factoid
The cubesat design standard was devised in 1999 by professors Jordi Puig-Suari of the California Polytechnic State University and Bob Twiggs of Stanford. A basic cubesat measures 10 centimeters on a side, but designers can join multiple units together to form 2U, 3U cubesats, all the way up to 27U. To release the cubesats in orbit, Cal Poly designed the Poly-Picosatellite Orbital Deployer, or P-POD for short. This lightweight metal box attaches to an expendable rocket’s upper stage to isolate the cubesats from the primary satellite and eject the cubesats in orbit after the primary satellite has launched. Houston-based NanoRacks produces a deployer to launch cubesats from an International Space Station airlock.
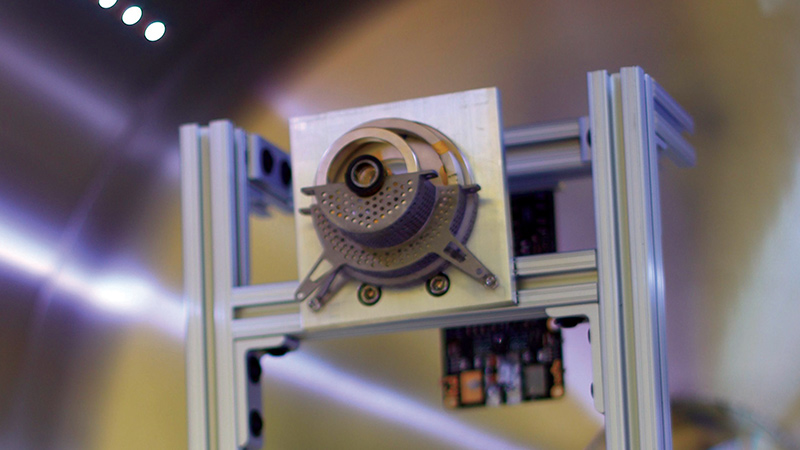
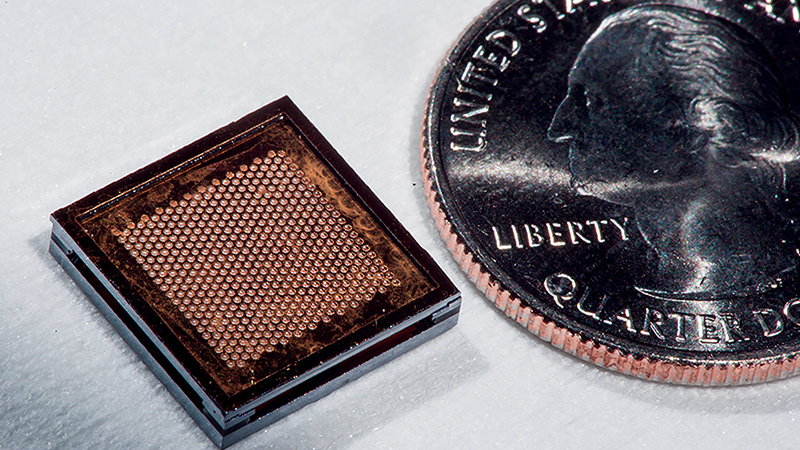
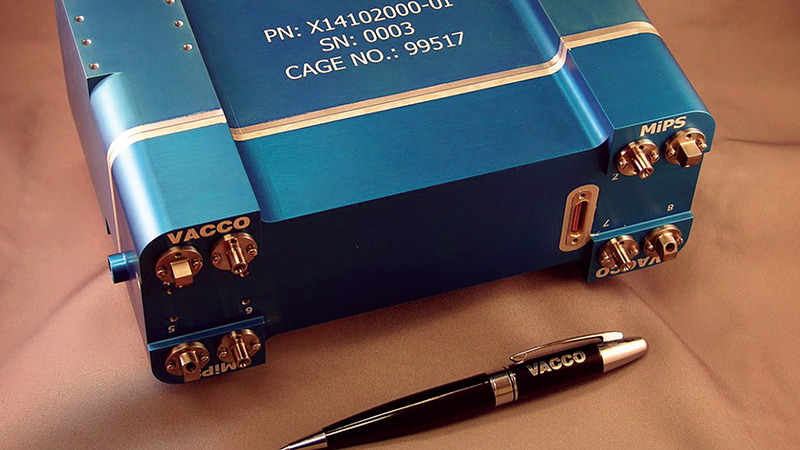
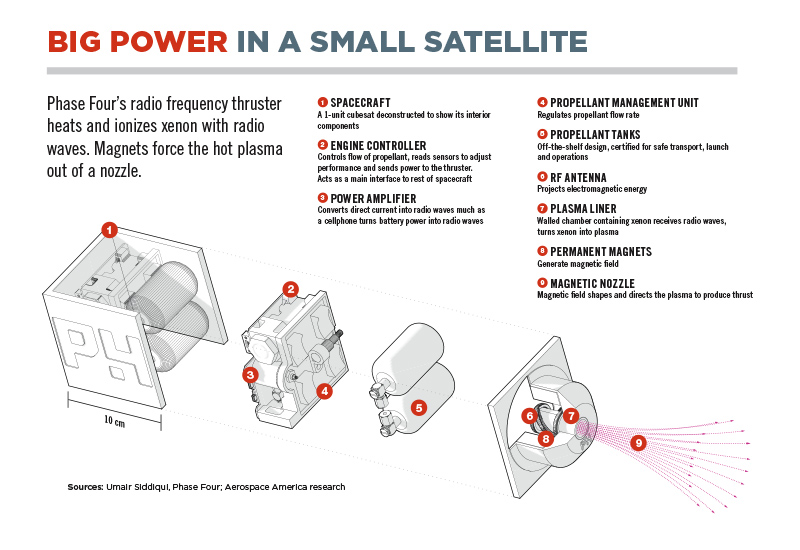