Beating Curiosity
By Leonard David|September 2017
A next generation robotic rover could start rolling across the Mars surface in 2021
Once NASA’s car-sized Mars 2020 rover reaches the surface, it must roll about the same distance in two Earth years as the Curiosity rover has traveled in five. NASA wants it to zip, in rover terms, from one scientific feature to the next. It must do this without the excessive wheel wear that has slowed Curiosity’s journey. It also must do something Curiosity was not equipped to do: drill solid samples from Martian rocks with a device called a corer; deposit the samples in tubes and drop the tubes on the surface for a future explorer, probably another robot, that would send them to Earth. Scientists would scour those samples for evidence of ancient life such as microbes. Much of that science hinges on improved navigation during the landing, so the rover can dare to land somewhere even more interesting than Curiosity’s site.
Here is the catch. The NASA-funded Jet Propulsion Laboratory in California must achieve these goals while staying as close as possible to the heritage design of Curiosity. That’s the only way managers will be able to stay within the project’s $2.4 billion estimated price. Months of testing lie ahead that should tell us whether they have taken the right steps to wring the most out of the new rover.
The blueprint passed its critical design review in February, and the first components are arriving at JPL, where personnel will build Mars 2020. Subsystem development continues along with testing of engineering models. All this is in preparation for rigorous system level tests beginning in 2019 ahead of the planned 2020 launch in July or August.
Daredevil landing
In most but not all ways, getting the rover to the surface will be like Curiosity’s “seven minutes of terror,” as engineers dubbed the plunge from the top of the Martian atmosphere to the surface.
Here’s what will be the same: The Mars 2020 descent will begin with the spacecraft dead reckoning its initial position and velocity from the transit time of radio signals from the Deep Space Network, followed by data from an onboard inertial measurement unit. At this point, the spacecraft consists of the rover and the Sky Crane landing apparatus tucked inside an aeroshell consisting of a heat shield and backshell. The heat shield must protect Mars 2020 from temperatures that will soar to 2,100 degrees Celsius due to atmospheric friction.
Closer to the surface, a parachute will deploy and the heat shield will drop away to expose the rover-Sky Crane assembly and provide a clear view of the surface for a radar.
Seconds later, onboard software will start calculating higher fidelity velocity and altitude from the radar data to determine when to fire up the vehicle’s eight retrorockets.
Before those rockets fire, the backshell and parachute must separate, leaving the rover-Sky Crane assembly in free fall temporarily. The rockets then fire to slow and maneuver away from the falling backshell and parachute.
Within meters of the surface, the Sky Crane will unspool the rover via an “umbilical cord” and bridle. After touchdown, pyros will cut the ropes and umbilical cord so the Sky Crane can rocket away and crash a safe distance from the landing site. Mars 2020 will be ready to roll.
Here’s what will be different:
NASA wants the rover to touch down “close to the fun stuff,” meaning scientifically interesting features, says JPL’s Allen Chen, the lead for the Cruise and Entry, Descent, and Landing Phase. “If we have to spend the entire lifetime of the rover just driving to get to the first sample site, that’s not good,” Chen says.
Even a few kilometers of accuracy will matter in the landing, because Mars 2020, although faster than Curiosity, will still reach speeds of only tens of meters per hour. So NASA has tightened the landing ellipse around the target from Curiosity’s requriement of 25 kilometers by 20 km to 16 km by 14 km for Mars 2020.
Daring to set the rover down near this “fun stuff” will mean diverting around large rocks, cliffs, scarps or other slopes.
Taken together, the goal of tightening the ellipse and taking on difficult terrain will require two techniques that Curiosity was not equipped for.
One is called Range Trigger, and it’s intended to shrink the ellipse by triggering the parachute deployment based on the location of the aeroshell relative to the surface. By contrast, Curiosity deployed its parachute when a particular velocity was reached.
The beauty of Range Trigger is that it will require no additional sensors, Chen says. It will crunch the inertial readings that already would be collected in the heritage design to determine where the aeroshell is relative to the surface. “Based on the mass of the vehicle and the predicted aerodynamics, we have a pretty good idea how far the spacecraft will travel between parachute deploy and landing,” Chen explains. In fact, curiosity “knew” it was slightly beyond the desired parachute deployment point when it released its parachute, Chen says.
The other new technique is terrain relative navigation or TRN. It’s why scientists feel emboldened to land amid challenging terrain.
After heatshield separation, cameras will photograph the surface as the aeroshell descends under the parachute. The TRN software compares those images to a stored digital map created from images taken by NASA’s Mars Reconnaissance Orbiter. If the rover is headed for a hazard, the software identifies a safer spot on the map and steers the rover and Sky Crane assembly there once the parachute and backshell are jettisoned and the rockets are firing.
To put it simply: “Terrain relative navigation gives us the ability to dodge things,” says Chen.
Scientists have agreed on three possible landing sites for Mars 2020, and Chen says “we feel comfortable going to any of the three selected landing sites.”
NASA had planned to test TRN in the field next year on a helicopter under various lighting conditions and classes of terrain, but has bumped that to 2019. In similar airborne tests a few years ago, NASA evaluated the underlying math for TRN. “We have shown that the algorithms work and that’s kind of the magic sauce,” Chen says.
NASA also plans to gather sights and sounds during the descent phase this time. Cameras and microphones will record the craft’s parachute deployment, for instance. Once on the surface, microphones on the rover are expected to capture a variety of sounds, including the turning of the rover’s wheels, drill operations, perhaps even the winds of Mars. Aside from the intrigue the public might get, engineers also see this as a diagnostic tool to assess the performance of components.
Addressing technical risks
Working off the design of Curiosity has been a “blessing and a curse,” says Jennifer Trosper, the deputy project manager for Mars 2020. As a member of the Curiosity team a few years ago, she led the study group that determined the adjustments that would be required to the design of Curiosity, also known as the Mars Science Lab.
The blessing is that “we’re not sitting here with a blank sheet of paper like we were with MSL,” she says. As for the curse, Mars 2020 can’t be “fully heritage” largely due to the elaborate sample caching system. Toss in for good measure, the challenge of avoiding problems such as the small holes discovered in Curiosity’s wheels.
“The main thing we’re doing is not skimping on the verification program. We’re testing everything again with its new purposes, in its new environment,” she says.
So subsystem tests will be followed by system level tests and functional tests of everything required for cruise, entry, descent, landing and rover operations on Mars.
The wheel deal
Since Curiosity’s landing in August 2012, wheel wear has been discovered beyond the anticipated dings and dents.
“Everybody wants that fixed,” Trosper says.
Engineers will do that by making the skins of the Mars 2020 wheels about twice as thick as Curiosity’s (1.65 millimeters compared to .75 mm), and by streamlining the pattern on the treads, called grousers. “Essentially the grouser pattern on MSL allowed for stress concentrations we wanted to eliminate,” Trosper says.
It turns out that Curiosity’s auto navigation mode, which was tried early in the mission, might have contributed to the excessive wheel wear by steering the rover over small, pointy rocks.
Faster roving
Understandably, the fear of causing wheel wear “makes you not want to use the auto nav,” Trosper says of Curiosity. Indeed, controllers at JPL’s operations center now prefer to drive Curiosity in the ground-directed mode, which means sending fresh navigation commands daily. With the rover stopped, controllers receive images of the terrain ahead of its navigation cameras. Controllers can assess the landscape for perhaps the next 100 meters, but beyond that distance, the resolution of the images becomes too coarse to reveal obstacles. The next navigation commands are uploaded in a planning process that can take 10 hours.
The story should be different for Mars 2020. Trosper predicts that controllers won’t hesitate to drive the new rover in auto nav, because they won’t be afraid of causing wheel damage.
With its finer resolution navigation cameras and smarter auto nav algorithm, Mars 2020 will spot areas likely to have pointy rocks and avoid them.
These improvements are one reason managers expect Mars 2020 to zip, relatively speaking, from one science target to the next. Don’t expect wheels to be spitting dust and dirt airborne, though. Mars 2020 will cover up to 225 meters in three hours at speeds up to 75 meters per hour. Even if Curiosity could operate in auto nav, the best it could manage would be 15 to 20 meters per hour or 45 to 65 meters in each three hour span.
Still, for a rover manager like Trosper, Mars 2020’s speed amounts to putting “the pedal to the metal.”
Making this smarter auto nav possible required adding a second computer processor to Mars 2020 compared to Curiosity’s one. The additional processor freed up the main processor to perform navigation calculations, while the second processor will be dedicated to rapidly processing images from the rover’s navigation cameras.
Mars 2020 will do “image processing and driving at the same time,” also known as “thinking and driving,” Trosper says. By contrast, Curiosity’s auto nav software, when in use, runs in series to acquire images, process them, select a path and then drive.
The difference should be profound. If Mars 2020 spots a rocky obstacle it could decide to straddle it and drive over it. “MSL’s algorithm would throw up its hands and go, ‘I can’t find a path!”
It’ll take more than technology, though, to speed up the action on Mars. The Mars 2020 team wants to get the 10 hours of work required in the operations center down to five hours partly by having teams of operators working in parallel. NASA runs the operations center from 6 a.m. to midnight, so a shorter timeline reduces the odds that work will spill from one day to the next.
Weight watching
Achieving all this without busting the rover’s mass allowance has been a chore. The decision to add the second processor added some mass, but most of the mass growth so far comes from an increase in the expected mass of the instruments and the corer, the device on the turret at the tip of the rover’s 2-meter-long arm. Also, a camera called Watson, short for Wide Angle Topographic Sensor for Operations and Engineering, was added to the turret.
The NASA Office of Inspector General, which routinely audits agency projects, discussed the mass issue in a January report. At a preliminary design review in February 2016, project managers expected the rover’s mass would be 1,013 kilograms. The report notes that the estimate had grown to 1,041 kg, close to the rover’s 1,050 upper mass limit.
Trosper says all is “OK” right now on the mass issue.
In the final analysis, it could turn out that managers had no choice but to approach the mass limit for Mars 2020 if they were to outperform Curiosity. When it comes to navigation, “We absolutely have to spend our time in the selected regions of interest, looking at targets and deciding what to sample. We can’t spend our time driving,” Trosper says. And once at those targets, Chen’s fun can begin.
Ben Iannotta contributed to this report.
Related Topics
Space SciencePossible landing sites
Earlier this year, scientists spent several days narrowing a set of eight previously picked go-to spots for the Mars 2020 rover. The down-selection process was led by a Mars 2020 Landing Site Steering Committee, co-chaired by NASA and the Center for Earth and Planetary Studies at the National Air and Space Museum. Here are the remaining options:
JEZERO CRATER: Hosts a dried up Martian lake that might be a repository of past microbial life.
NORTHEAST SYRTIS MAJOR: A dormant volcano created almost entirely by lava flowing and cooling. This shield volcano abuts a huge impact crater and an area thought to be warm and wet long ago.
COLUMBIA HILLS/GUSEV CRATER: Previously surveyed by NASA’s Spirit rover, this site appears to be flush with sinter deposits from a long-gone hot spring. Similar sites on Earth are known to support microbial life.

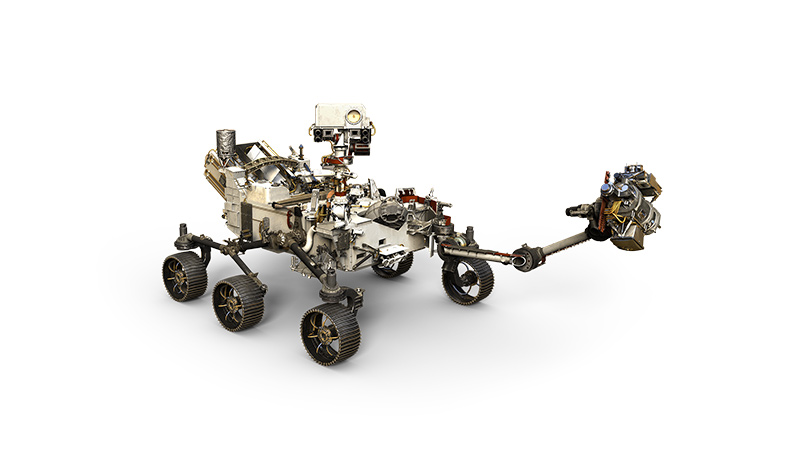