Robotic landing legs
By Keith Button|July/August 2017
Making it easier for helicopters to land in harsh conditions
On the fields of a former dairy farm in Greenville, Georgia, a pilot manipulated a helicopter remote controller as a group of students and engineers stood behind him. He steered the unmanned rotorcraft to a sloped patch of ground where no conventionally equipped helicopter would dare land. Four aluminum legs unfolded, and as each leg touched the uneven ground, a microprocessor commanded the joints in each leg to bend exactly as needed to keep the helicopter’s fuselage and main rotor disk level. The legs locked themselves into place and the helicopter paused for 20 seconds, then took off to repeat the sequence.
Professors, research engineers and grad students at the Georgia Institute of Technology built this robotic landing gear with funding from DARPA starting in 2012. They attached the legs to a Rotor Buzz 2 unmanned crop-dusting helicopter and conducted a series of test flights from 2013 to 2015. Now the Georgia Tech team is preparing to show how the technology could handle the rolling deck of a ship in rough seas.
Success at sea and over land could solve a vexing shortcoming of helicopters: They can’t land on or take off from a slope greater than 10 degrees. With steeper slopes, the main rotor disk tilts too much during takeoff or landing, which can cause sideways thrust while part of the landing gear is still touching the ground or deck of a ship. Those conditions can cause a rollover in which the helicopter blades spin into the ground, or deck or water.
Cracking the 10-degree barrier by keeping the fuselage level could open up new possibilities for flights from ships or into rugged terrain for medevac, humanitarian and military missions.
Even away from rough seas, it can be hard for a pilot to assess on the fly what angle a landing site will pose. “If you’re on an unprepared surface in an open field, you could land in that same area 10 times, and one time you land with zero slope and another time you may land getting the perturbations in the slope and you have a 14-degree slope that you fell into,” says Mark Costello, an aerospace engineering professor at Georgia Tech and head of the robotic landing gear program.
So far, Georgia Tech has proved that a 113-kilogram Rotor Buzz 2 can land and take off from slopes of up to 20 degrees. Ultimately, the team wants to show that the legs will do the same for rotorcraft of up to 3,200 kilograms, whether conventionally piloted or unmanned, without adding so much weight that helicopter makers will reject the idea.
A legged solution
Georgia Tech’s Costello has three operational concepts in mind for the robot legs: a kit that could switch out a helicopter’s traditional skids or wheeled landing gear for the legs; new helicopter designs with the robot legs as retractable landing gear; and futuristic ideas, such as a Humvee designed to carry a detachable helicopter that would be equipped with the legs. The kit concept is probably closest to realization because it is the least expensive and easiest to build option for helicopter manufacturers. With the kit concept, for some helicopter designs the legs will need to fold up on the sides of the fuselage, while for others they will fold up underneath, depending on the helicopter’s structure, attachment points, weight distribution and other factors.
The Georgia Tech team divided the robotic legs development duties into two groups: one for the leg structures and another for motion and control of the legs. For both groups, minimizing the weight of the robotic legs was a priority because the greater the weight, the more they cut into the cargo capacity of the rotorcraft.
The members of the structures group knew they needed to select materials, and at the right thickness and shapes, to build legs that were as light as possible while still supporting the necessary loads. They chose aluminum for the metal’s light weight and strength. Lightweight legs also provided the benefit of needing less power to manipulate, so the design team could also drive down one of the main contributors to weight: the battery size.
A basic problem for the motion and control group to solve was how to tell the onboard microprocessor about the slope of the landing surface: the elevations of the spots where the robotic feet would set down. LIDAR or other optical sensors were not precise enough to read the landing slope, so the group selected off-the-shelf pressure pads for the feet of the legs. These sense when the feet touch down and how much pressure they are exerting against the ground. With the information provided by the pressure pads, the microprocessor can decide when and how much to move each leg.
The motion and control group also needed to program the control logic for the legs to keep the helicopter level during takeoff and landing. The group came up with three options. One option was to direct the onboard microprocessor to continuously monitor pitch and roll as the helicopter landed. This logic would tell all four legs to move as needed to keep the pitch and roll angles at zero. The team also considered adapting the virtual-model control logic that designers of walking robots rely on.
They chose an entirely different approach for the Rotor Buzz flights. The control logic instructs the microprocessor to command the joints in each leg to bend freely as that leg touches down, once the pressure reading for that foot reaches one quarter of the weight of the helicopter. The microprocessor also coordinates the joints in each leg so that the foot does not move horizontally. This logic is simpler than the other two logic options, so it is less prone to glitches. The other two logic options would require either monitoring of pitch and roll readings while including those readings in a feedback loop to adjust the leg joints, or accessing the pre-set programmed movements of the virtual-model control logic. Plus, the two options would also need the same foot pressure pad readings as the Rotor Buzz flights control logic.
For the at-sea design, the team plans to test both the Rotor Buzz flight control logic and the option that continuously monitors pitch and roll. The engineers will save tests of virtual model control for future robotic leg designs.
In designing the individual legs, the Georgia Tech team knew it needed them to keep the fuselage level during takeoff and landing, and fold up during flight. The researchers chose the simplest design that accommodates both objectives: two joints, with one at the bottom of the fuselage where the leg attaches to the helicopter, like a hip, and one about halfway down the leg, like a knee. The team had an easier time writing the control logic software for two joints per leg than they would have for legs with more joints, plus the legs weighed less with only two joints. Electric motors drive gear boxes in the joints. These gears bend each leg at the two joints while keeping the feet moving straight up and down only, because any sideways motion by the feet could tip the aircraft. With a ground landing, when all four legs have made contact, a brake on each joint locks them in place with the helicopter resting in a level position. The brake is released only when power is applied, so that any power failure will leave the leg joints rigid.
The team needed the robotic legs unit to be self-contained to avoid the complication of connecting it into a helicopter’s power system, avionics and flight control computer. For power, they chose lithium ion batteries. For larger helicopters, such as traditionally piloted models, power for the legs will come from the aircrafts’ main hydraulic systems.
For the helicopter tested in Greenville, each segment of the legs — from hip to knee and from knee to ground — was about one-third of a meter long. As the legs are scaled up for larger aircraft, each fully extended leg will measure about the same length as the individual helicopter’s fuselage ground clearance with normal landing gear. Other helicopters may swap the four-legged system for three-legged versions, or variations on the two-jointed legs. As the robotic legs are scaled up for larger and larger helicopters, the legs and batteries to power them will take up roughly 7 percent of the maximum payload, Costello says. For a 2,700-kilogram manned helicopter, the robotic legs landing gear would weigh about 90 kilograms.
Sea legs
In May, the engineers built legs like those they hope to take to sea and tested their durability in the university’s lab. They attached them to a frame to simulate a helicopter’s size and weight, locked the leg joints, hoisted the frame and dropped it. They started these hoist drops from a few centimeters off the ground and worked up to 1.2-meter drops. Demonstrating that the landing gear is durable assures the team that legs will hold up and protect the helicopter in a hard-landing scenario, such as in an emergency landing or if the aircraft loses vertical thrust.
Starting in November at an Arizona test site, the Georgia Tech team will test robotic legs on an unmanned helicopter weighing about twice as much as the Rotor Buzz 2. The testing is funded by an airframe manufacturer that Costello declined to name and by DARPA.
The team will attach the legs to the helicopter and try to land it on a platform that pitches and rocks to simulate the movements of a ship at sea. Next year, they will take the helicopter to sea to test takeoffs and landings on a ship. For manned helicopters, landing on a ship’s deck in high seas is one of the most dangerous maneuvers that a pilot can attempt.
So far, computer simulations show that the robotic legs should be able to set the helicopter down on a ship deck under weather conditions creating waves 4 to 6 meters high, Costello says. The legs would keep bending as the deck pitches and rolls, if necessary, to keep the aircraft level while sitting on the deck, before a crew straps it down to the deck, or during takeoff.
Future landings
Robotic legs make the most sense for new and evolving types of helicopters, says Ashish Bagai, a program manager at DARPA who worked with Costello in the early days of the robotic landing gear project. Bagai says he wouldn’t advocate putting legs on a 5,500-kilogram helicopter, because the weight of the legs would cut too much into cargo capacity. But for rotorcraft weighing up to 3,200 kilograms with specific missions, robotic legs or maybe landing gear kits that could swap out regular landing gear for the legs would open up new mission possibilities. “What’s important is that you apply it to those types of air vehicles that you wish you could do certain things with today but you cannot,” Bagai says.
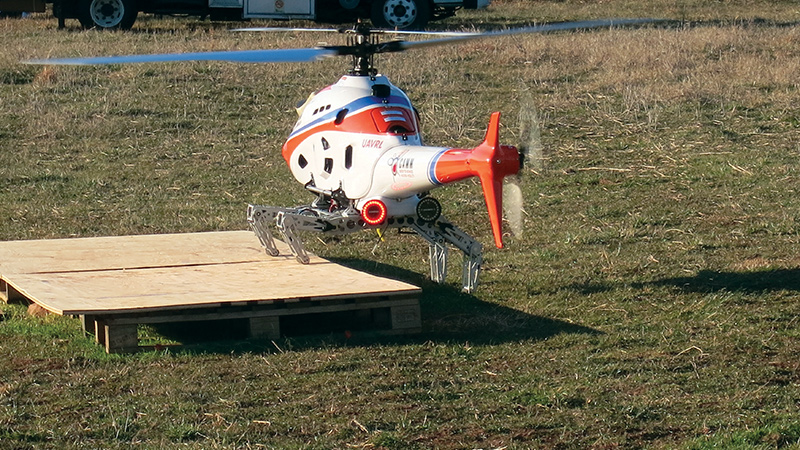
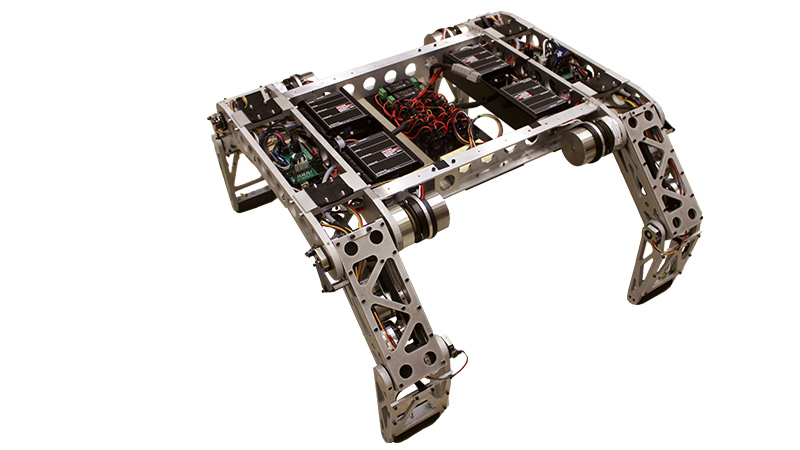
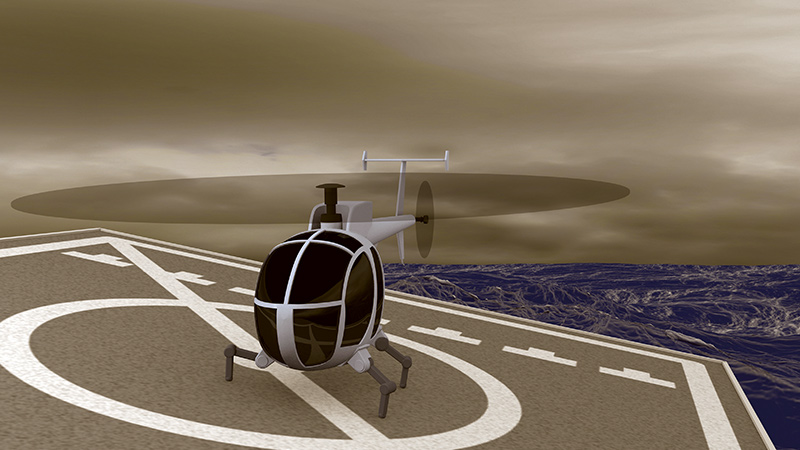