Stay Up to Date
Submit your email address to receive the latest industry and Aerospace America news.
Fielding a hypersonic missile that can cull oxygen from the air for propulsion would mark a dramatic turn in the U.S. and allied race to master hypersonic weaponry. Crafting such designs has proved challenging largely because the physics are not well understood and designs can’t be easily tested in wind tunnels. Keith Button spoke to researchers who think they have a solution.
On its way to a target, an air-breathing hypersonic missile would need to plow through air of varying densities, temperatures and chemistries, compressing the air by forcing it into a narrow passage to provide oxygen for combustion. Scientists know from the brief experimental flights so far that the interactions with the air can result in strange phenomena. At about Mach 7, for instance, the bow shock from such a missile would begin to ignite the air in front of it, and by Mach 10, the air would be fully ignited.
Predicting how particular hypersonic designs will fare in such conditions has proved challenging, partly because of the limits of wind tunnel testing on physical models. Hypersonic flows can generally only be achieved for fractions of a second due to the huge tanks of air required, and even then, scientists have more than a need for speed. They need to know if control and combustion can be maintained in the thinner, colder, calmer air at high altitudes and in the thicker, warmer, more disturbed air at lower altitudes, for instance. The trouble is that some wind tunnels have particular strengths, and no single wind tunnel test anywhere can replicate all the characteristics of the medium. So successions of tests must be run.
“Whenever you check one box, another box gets unchecked,” says hypersonics researcher Carlo Scalo, an associate professor of aeronautical and astronautical engineering at Purdue University in Indiana. The unchecked box requires a separate test, sometimes at a different facility.
These limits are one reason why air-breathing hypersonic missiles have yet to be fielded by China, Russia or the United States, despite the military value of speeding faster than traditional cruise missiles without having to initially fly to suborbital space, as boost-glide hypersonic missiles must.
Of special concern are the controllability of such a missile and the performance of the flow path. Firing up a supersonic combustion ramjet, or scramjet, and keeping the engine lit is like holding “a candle in a hurricane that’s about 10 to 15 times faster than the fastest hurricane you can imagine,” says Anand Veeraragavan, associate professor and co-director of the Centre for Hypersonics at the University of Queensland in Australia.
The solution, a team of U.S. Navy-funded researchers say, could lie in developing a single computational model that accurately reflects key characteristics of the air flow, including its density, chemistry and temperature, so that performance of a scramjet design can be predicted. Such a model would reduce but never eliminate the need for wind tunnel testing.
“It’s impossible to make a computational model that will perfectly represent reality,” says Venkat Raman, a professor at the University of Michigan and the lead principal investigator for the project, “Discovering and Modeling Turbulence and Chemistry Interactions in High-Speed Reactive Flows.”
Last year, Raman assembled a team of experts in modeling, molecular chemistry of combustion, scramjet engine design and hypersonic wind tunnel testing to pursue development of the computational model. In September, the team won a $7.5 million grant from the Office of Naval Research to pursue its development for five years ending in 2026.
Divide and conquer
The “one big model,” as Scalo calls it, would need to be created from separate models focused on the specific characteristics of the hypersonic flow — the boxes that need to be checked, in Scalo’s analogy.
An obvious challenge is that air flows externally around an air-breathing vehicle but also through it. Burning fuel in the engine creates chemical reactions between the hydrocarbon molecules in the fuel and the nitrogen and oxygen in the air, so detailed models of those reactions and their release of heat would need to be incorporated into the internal flow model. Meanwhile, modeling the external flow would have to account for a different set of chemical reactions: The high temperatures at hypersonic speeds can cause oxygen and nitrogen molecules in the air to break down or chemically react with the surface of the missile.
So the professors and their respective teams of post docs and graduate students split into three groups that will nevertheless coordinate closely: a group dedicated to the external flow, a group devoted to the internal flow and propulsion, and a group of mathematicians to help both the external and internal modelers.
“The groups of people that have been looking at these two flow paths have been developing over decades different expertise,” says Scalo. “The goal of this grant is to bring them together.”
By the end of the five-year project, which is one of the Multidisciplinary University Research Initiative grants that the U.S. military services award annually, the external and internal flow teams aim to have developed shared mathematical tools to build their models with, Scalo says.
As Raman explains, “in order to separate signal from noise — physics from just uncertainties in models and experiments — we need a mathematical framework. This is what the team is doing — using a novel mathematical framework to develop new tools and experiments that can probe the scramjet at extreme resolution.”
The external engineering team has already received a boost from University of Southern California mathematicians, applying advanced mathematical tools to some of their simple external flow models to improve their accuracy, Scalo says.
“That’s something that engineers cannot do without mathematicians,” Scalo says. “Mathematicians have these magic tools to do that.”
One of them is polynomial chaos expansion theory. Take, for example, any computational model that has a few “fudge factors” built in — variables that humans change the settings for based on their experience of what has produced the best results in the past. The polynomial chaos expansion theory tool can change the settings for hundreds of such fudge factors at the same time and provide a prediction of the best setting, or predict how much each of the fudge factors might influence the model’s outcome, Scalo says.
To build a computational model for the exterior flow, the professors have further divided the task: Raman’s Michigan team is creating a general model that can encompass the entire surface of a missile, while Scalo’s Purdue team is making one to home in on a napkin-sized piece of the surface. The detailed model, focusing on air flow within 2 millimeters of the surface of the missile, divides the space into a grid of calculations for cubes that are 10 or 20 microns wide. The grid — also known as a mesh — for the general model will calculate for cubes that are 100 to 1,000 times larger, with pockets of finer grids embedded within it.
“He gets the whole solution, but not very accurately; I get a very accurate solution, but of a small portion of the flow,” Scalo says. The two teams intend to merge their computational models.
Accounting for shockwaves
For both the external and internal flow teams, their computational models will have to account for shockwaves that form when a component of a vehicle, such as the engine inlet on a missile, pushes through the air at hypersonic speeds. Shockwaves introduce sharp changes to air pressure, density and temperature, which are all critical in determining aerodynamic characteristics such as lift and drag. Plus, shockwaves can cause turbulence and other sudden changes in the direction of flow, which can affect the stability of combustion in an engine or how well a missile flies.
Unlike subsonic air flows — the realm of most conventional aerodynamic models — at hypersonic speeds the air can be highly compressible and squishy. So when the air temporarily slams to a halt at the surface of an object in the flow path, the air upstream from the object squishes down into a thicker substance and a shock develops, with air pressure jumping as much as 10 times higher, depending on the velocity.
The high temperatures created by hypersonic air flows also change the aerodynamics. At between Mach 5 and Mach 10, the air is heated to up to 2,700 degrees Celsius — so hot that the skin of hypersonic missiles must be made of a ceramic or ablative material because a metal exterior would melt. Above Mach 10, as temperatures exceed 3,700 degrees Celsius, the air is set on fire even before it touches the missile, and then other chemical reactions are set off when it does touch the surface. The combination of heat and pressure causes oxygen and nitrogen molecules in the atmosphere to break apart, creating their own fuel-oxidizing mixture.
Before the current five-year modeling project, Scalo had developed aerodynamic models for low hypersonic speeds based on supersonic models. Under the current grant, he is expanding the models into higher velocities at which the heat and pressure extremes create chemical reactions that alter the aerodynamics.
Bullet launch
In Australia, a close ally of the United States, Scalo will put his computational models to the test at one of the wind tunnels in Queensland. He’ll focus specifically on high-density flows that would ignite in front of a vehicle, conditions that the Queensland tunnel can replicate.
“Great, that checks a box that cannot be checked at Purdue,” he says, referring to his university’s tunnel that is not involved in the project but is known for its exceptionally smooth flow replicating calm air at higher altitudes. He’ll compare his model’s aerodynamic predictions against tests of a physical model and then adjust the computational models based on the test results.
For each of those tests, a tank of high-pressure air will provide the propulsion to launch a 90-kilogram piston down a long tube, like a bullet through the barrel of a gun, compressing air in front of it until a metal disk 1 to 2 millimeters thick ruptures, with the thickness determining the size of the shockwave caused by the rupture. The rupture will push air through a nozzle into a 10-meter-long shock tube containing the mock vehicle shape, over which the hypersonic air flows for a split second. With readings from pressure sensors affixed to surfaces in the shock tube, the Queensland team can calculate the velocity and temperatures created during the test.
Scalo plans to begin adding simple chemistry models to his computer model and then progressively more complicated chemistry models until his computer model fully matches the wind tunnel tests. Then he’ll consult with the internal flow engineering team to check his modeling of chemical reactions against theirs.
“The bet is that we will be able to find the same type of physics — the interaction between flow and chemistry,” he says.
For the internal flow team, one of the main tasks is to build a combustion chemistry model. If the combustion chemistry model is wrong, for example, then the predictions by the larger computational model for the internal flow about how easily the scramjet engine will fire up will be inaccurate. To improve the accuracy of potential chemistry models of hypersonic engines, part of the team is checking the models against 1,400 sets of data created by tests dating back to the 1920s, says Hai Wang, professor of mechanical engineering at Stanford University.
The professors need to incorporate data from a wide range of these previous experiments into their combustion chemistry model to account for a wide range of conditions in a scramjet engine, including shockwaves that create air pressure ranges from normal at sea level to 100 times greater than normal, Wang says. These tests include shock tube experiments with lower than hypersonic flows but which created sudden air pressure changes that heated gas up to combustion almost instantaneously.
Inside a scramjet engine, there are intentionally designed cavities that slow the air so that it can be ignited and areas where the air will momentarily stop, which dramatically increases its temperature, says Tonghun Lee, professor of mechanical science and engineering at the University of Illinois Urbana-Champaign. For instance, the temperature of air that was traveling at Mach 5 can suddenly rise to 1,000 degrees Celsius.
As a starting point for modeling this internal flow phenomena, Lee turned to gas turbine engine models, which will be tested in hypersonic conditions to see how their predictions hold up. But those turbine engine models have been meticulously designed and tweaked for maximum accuracy for a very specific range of temperature and pressure and for a predictable set of circumstances: Air comes in, it compresses to a high pressure, and then fuel is added and ignites. The hypersonic model has to make predictions over a much wider range.
To make sure a model can do that, plans call for running hypersonic air flows through mock engines at Illinois Urbana-Champaign wind tunnels. Windows in the mock engine ducts will expose the flow path to sensors to record the chemical reactions, molecular states of air and fuel, temperatures and velocities at every point in the flow path, says Lee, who will head up the wind tunnel testing. High-speed cameras capable of shooting 100,000 frames per second will record images as lasers scatter light off titanium oxide particles added to the flow stream for particle image velocimetry. As successive images of the 40-nanometer-diameter particles are recorded during the test, their velocity will be calculated from their movement.
If things go as Raman hopes, the impact of the project will be bigger than any particular application.
“The scope here is more fundamental. There are certain things about the physics of these machines that we do not understand,” he says.
Record-setting flight
In March, a Hypersonic Air-breathing Weapon Concept missile powered by an air-breathing supersonic combustion ramjet, or scramjet, “set the U.S. record for scramjet endurance,” DARPA director Stefanie Tompkins told a U.S. Senate Armed Services subcommittee. The missile was the Lockheed Martin version of the HAWC, and it was dropped from a carrier aircraft and boosted by rocket to the scramjet speed envelope. The scramjet then lit and accelerated the missile at hypersonic speeds, according to DARPA. The duration of the scramjet portion of the flight and the top speed were not specified, but DARPA said the missile flew under scramjet power for longer than 210 seconds, the record held by the U.S. Air Force’s X-51A. — Keith Button
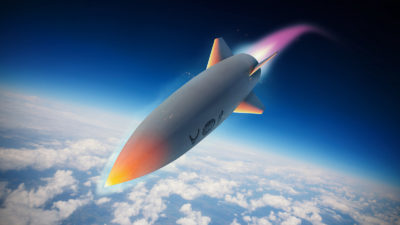
“The scope here is more fundamental. There are certain things about the physics of these machines that we do not understand.”
Venkat Raman, University of Michigan

About Keith Button
Keith has written for C4ISR Journal and Hedge Fund Alert, where he broke news of the 2007 Bear Stearns hedge fund blowup that kicked off the global credit crisis. He is based in New York.
Related Posts
Stay Up to Date
Submit your email address to receive the latest industry and Aerospace America news.
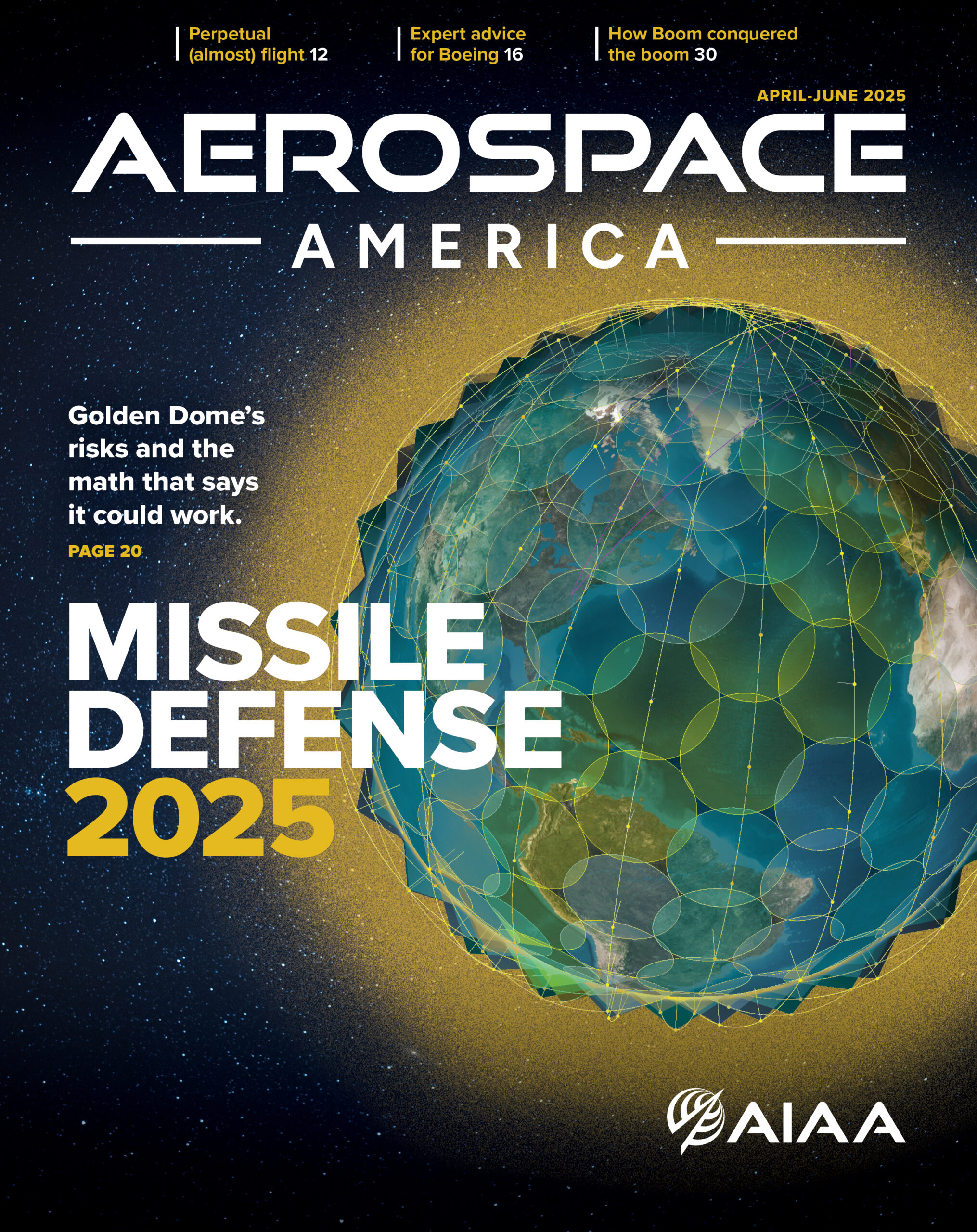
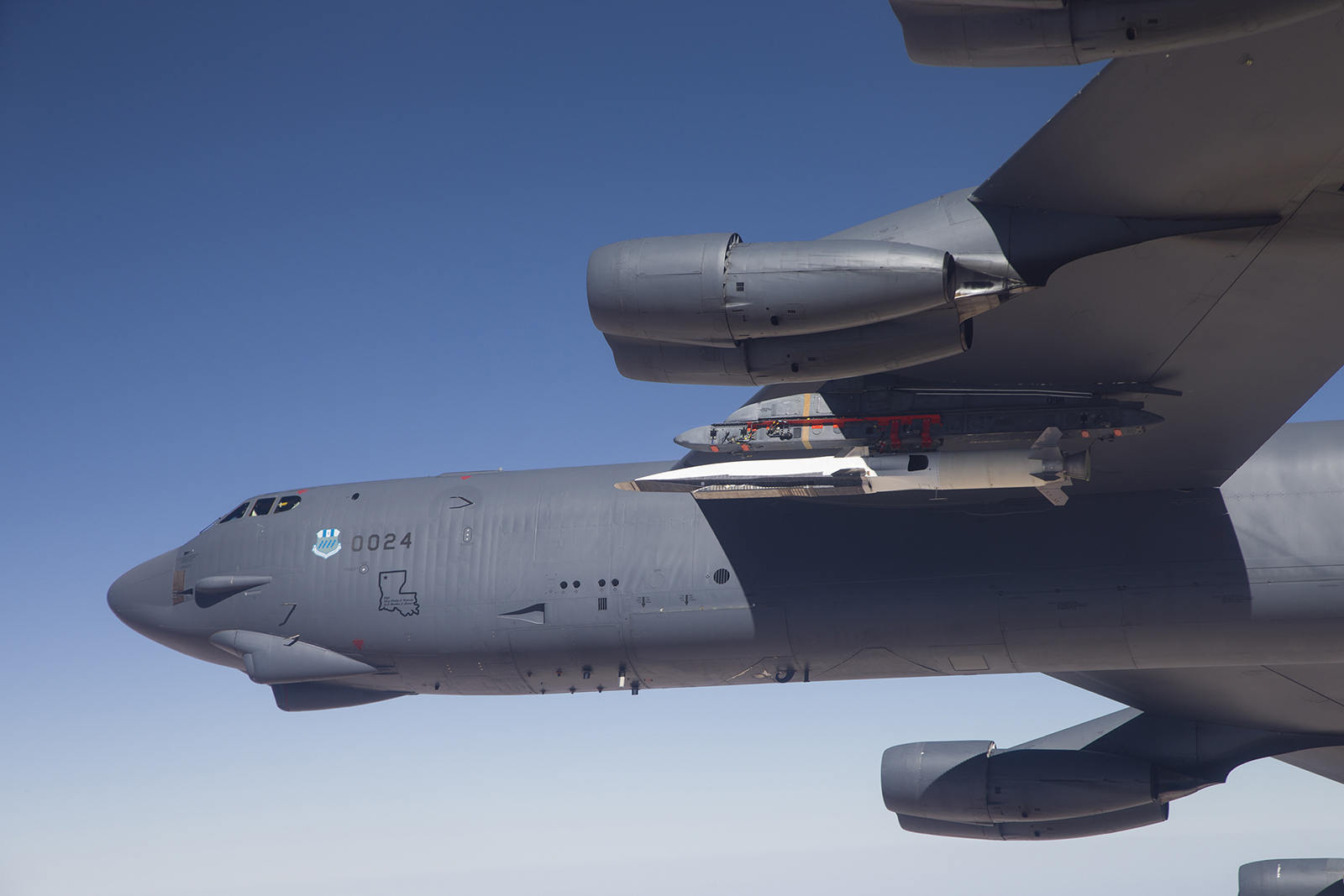