Nuclear nonsolution
April 2018
Detonating a nuclear bomb is unlikely to protect Earth from a far-off asteroid or comet headed our way
Detonating a nuclear bomb sounds like a common-sense way to protect Earth from a far-off asteroid or comet headed our way. The reality is that the attempt would probably make matters worse, which is why it has been relegated to being a fringe idea. Planetary Astronomers Robert L. Marcialis, Nadine G. Barlow and Larry A. Lebofsky explain.
The community of researchers who worry about the issue of dangerous near-Earth objects on a daily basis long ago considered and wisely discarded the nuclear option that is favored in the opinion article, “Earth’s Best Defense,” in the February issue of Aerospace America. The reasons for this rejection had nothing to do with political correctness and everything to do with analysis, physics, and information shared through a series of Planetary Defense conferences held every two years. Allow us to explain.
Suppose an asteroid, 10 to 50 meters in diameter, were on a collision course with Earth. This is the size of the object referred to in the February article and that created the 1.2 kilometer-wide Barringer Crater near Winslow, Arizona. Such an object would be large enough to cause extensive damage, ballistically throwing ejecta as far as 3 km from the crater.
Let’s look at the content and energy of the Barringer object. Made of iron and nickel, it did not vaporize entirely on impact despite megaton estimates for the released energy of up to 60 MT. Many chunks were strewn about the surrounding countryside; many are now in collections. Those observations agree with the results of laboratory studies and numerical modeling that demonstrate how difficult it is to vaporize iron or mixes of iron and nickel. It’s therefore incorrect to expect that a nuclear detonation of similar megatonnage could vaporize such an object before it reached Earth.
Instead of neutralizing the threat, we would be left with a fragmented, disrupted body. True remediation is more likely if the target is not disrupted, but given a gentle, long-lasting nudge in a controlled manner. Fragmentation would compound our problems enormously. Instead of having to deflect one bullet, we would have a hailstorm of particles, large and small, to dodge.
Perturbation of each particle from its original orbit cannot be predicted, and we would have to ensure the entire ensemble misses Earth so as not to compound the damage inflicted. Note that a 10-centimeter wide iron fragment (the size of your fist) can survive atmospheric entry to hit the ground, heating and producing shock waves in the atmosphere on its way down. The 2013 Chelyabinsk bolide (estimated to be initially 20 meters in diameter) attests to how seriously damaging these shock waves can be.
Fragments of this body would be imparted with a range of kinetic energies and would drift apart. If detonation occurs at 1 AU and 100 days before Earth encounter, the cloud would have expanded to 17,000 km, or 1.35 Earth diameters. To ensure that this cloud misses Earth, we would have to ensure a deflection of at least 2.6 Earth radii (the radius of the cloud, plus the gravitational radius of Earth), plus a 50 percent safety margin, or nearly 4 Earth radii.
Dispersal also would occur along the trajectory, not just normal to it. A single impact here on Earth would become a string of impacts lasting more than an hour.
Note that a near miss is not acceptable risk. The threat avoidance problem is much more complicated. Here’s why: If the mutual orbits of Earth and the object intersect at one point in time, they will intersect at other times in the future. Fragments will continue to disperse even after the Earth encounter, becoming an ever-widening debris cloud. Tidal forces (the tendency of objects in a gravity gradient to drift apart with time), the Yarkovsky effect (the slight thrust caused by solar heating and re-radiation of thermal IR by the evening hemisphere), radiation pressure, and perturbations by other bodies of the inner solar system will constrain what constitutes a successful change in trajectory. Each of these stochastically adds to what comprises an acceptable “miss.”
Metallic bodies actually comprise only a small minority (about 4.5 percent) of the population of interplanetary bodies, as shown by the distribution of meteorites recovered from Antarctica. Much more probable is that the threatening body would have a stony composition. From the sample of asteroids and comets visited by spacecraft, representative porosities of these bodies are a few tens of percent. These bodies are most likely agglomerations of chunks, large and small. Any impulsive change in momentum is likely to fragment the body rather than deflect its trajectory, because it is mechanically weak.
There is overwhelming evidence that the average object is mechanically weak. Consider Comet Shoemaker-Levy 9’s impact with Jupiter in 1992. The comet was torn into 21 fragments merely by tidal forces in a previous encounter with Jupiter. For stony objects, including most asteroids and all comets, the “rubble pile” description is most appropriate.. Gravity and mechanical strength are close to zero. If you push a bucket of sand, it moves as a whole. Not the case for a sand castle. Should a stony object be headed our way, it is likely a wash between the kinetic impactor and a nuclear detonation: either would simply fragment the body.
Whether an object is stony or metallic, a failed first attempt at deflection by a nuclear device in all probability eliminates any chance of success on subsequent tries, even should sufficient time exist. The debris from the first attempt would produce what amounts to a field of kinetic energy weapons around the body. At an approach velocity of 11 km/sec, impact on the delivery vehicle by a rice-sized particle would be catastrophic to the vehicle and the odds of mission success.
Our best path to success depends on early detection and cataloging of potentially hazardous bodies, combined with knowledge of the physical properties of such a body, both keys to designing a reasonable course of action.
Here’s a sampling of the many methods of gentle diversion that have been proposed over the last couple of decades:
- Sending a massive satellite close to the body as a “gravity tractor” to pull the threat in a calculated and controllable direction.
- Landing a similar satellite on the surface of the body and pushing the threat with high specific impulse, low thrust rocket motors in the desired direction.
- Physically painting parts of the body’s surface with reflective and/or absorptive coloring.
- Orbiting a satellite equipped with lasers, which would vaporize select regions on the surface of the body, producing an in situ thrust.
These are just a few of the ways we can intervene. The reality is that the nuclear option is no option at all.
A more detailed version of this article is available here.
Robert L. Marcialis is a visiting scientist at the University of Arizona’s Lunar and Planetary Laboratory in Tucson and an AIAA senior member. He holds a doctorate in planetary science from the University of Arizona and a Bachelor of Science in aeronautical and astronautical engineering from MIT. Email umpire@lpl.arizona.edu
Nadine G. Barlow chairs the Department of Physics and Astronomy at Northern Arizona University in Flagstaff. She holds a doctorate in planetary sciences from the University of Arizona. Email nadine.barlow@nau.edu
Larry A. Lebofsky is a senior education and communications specialist at the Planetary Science Institute in Tucson, Arizona, and an AIAA senior member. He has a doctorate in earth and planetary sciences from MIT. Email lebofsky@lpl.arizona.edu
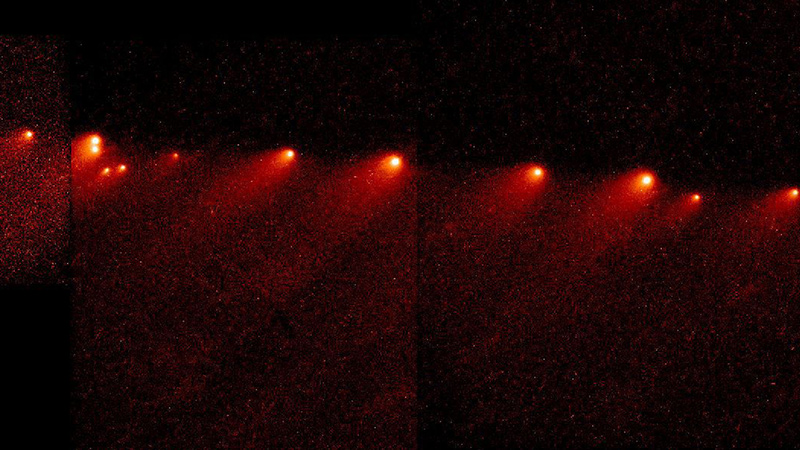