Containing a blade-out
By Keith Button|July/August 2018
NASA has long been working with the FAA and the aviation industry to improve technologies for containing broken fan blades and preventing damage like that which killed a Southwest Airlines passenger. Keith Button looks at a research project that could help prevent future tragedies.
When a fan blade snaps off inside a turbofan engine, as happened on a Southwest Airlines flight in April, a ring of metal or composites must keep the broken blade inside the engine. The engine rapidly winds down, shaking violently like a supersized clothes washer with an unbalanced load.
The broken blade stayed inside the engine in the Southwest incident, as it was supposed to do, but other debris nevertheless pierced the cabin of the Boeing 737-700, depressurizing the cabin and killing a passenger.
Even before the Southwest accident, researchers at NASA Glenn Research Center’s Ballistics Impact Lab in Ohio, were assessing the ability of new materials to withstand projectile impacts, including from broken blades, and chronicling the amazingly rapid progression of events when there is damage.
The work has been playing out under a five-year High Energy Dynamic Impact project that wraps up in September 2019, with funding from the Advanced Composite Consortium, which includes NASA, FAA, aircraft and engine manufacturers, and universities.
The archives of these test results will be fed into computer models to help airplane and engine designers more accurately predict the performance of their designs for blade-out containment and fuselage protection. The testing should also give the FAA more confidence in the accuracy of the models, which in some cases might substitute for certification testing.
With most materials, the ability to hold up under certain types of loading is predictable and well-known. But predicting the results of an impact, even on materials with well-known properties, is extremely complicated, says Mike Pereira, head of the Ballistics Impact Lab.
“It’s surprisingly difficult to predict penetration, even in simple metals,” Pereira says. “If you were trying to predict if a metal projectile will penetrate a flat panel of aluminum, your prediction is probably not going to be accurate.”
Once a material is damaged, it’s also difficult to predict whether it will maintain its strength and still do the job that designers intended it to do, Pereira says. Adding to the challenge, composite materials can be considered as miniature structures: Some have fibers running in different directions in each layer, for example. This structure makes it difficult to predict whether or how far damage will propagate through the material.
Pereira and his team get at these and other questions by firing gas-powered guns at materials. They have about a dozen gun barrels, ranging from 46 centimeters to 12 meters long and from 1.6 millimeters to 40.6 centimeters wide.
In addition to pieces of fan blades, the ballistics lab has shot sharp and blunt objects for impact testing, ranging from ice crystals to artificial birds made of gelatin containing micro-balloons to create specific desired densities.
Aircraft engine designers hire the ballistics lab to test their blade-out containment designs. The lab’s cost of testing a blade-out projectile is typically a few thousand dollars. “We test concepts relatively inexpensively and do multiple tests within a week, whereas a whole blade-out is a really expensive proposition. You destroy an engine,” Pereira says. To simulate a blade out, the containment ring, or containment case, is removed from an engine. These rings are typically about 60 centimeters wide, and they circle the fan blades. Safety regulations require that the case be wide enough to cover 15 degrees fore and aft from the center hub of the fan blades.
Until the 1970s, these rings were solid metal designed to withstand and deflect blade-out shrapnel so it wouldn’t penetrate the cowling, which is the outer shell of the engine, also called the nacelle. This metal “hard-wall” approach makes the engine heavy, so engine makers started developing cases that would permit broken blades to poke through the interior walls into an outer layer of Kevlar, inside the cowling. These “soft-wall” containment cases reduced the weight of the engine.
Today, more engine designers are adopting composite cases. The CFM56-7B engine — the engine on Southwest Flight 1380 that broke apart after a blade out, killing a passenger — has a hard-wall metal containment design. The Honeywell HTF7500 engine on Gulfstream G280 business jets is an example of a composite soft-wall containment design, and its composite and fabric materials were tested in Pereira’s lab.
The hard-wall and soft-wall designs each have pros and cons. With a soft-wall, the Kevlar must have room to stretch when impacted, and that means there must be adequate room for this expansion between the ring and the cowling. Designing in this empty space makes the engine’s diameter a bit larger, and that increases drag. The advantage is that the broken-off fan blade is captured away from the flow path, where other blades are spinning, so there are fewer secondary impacts with those blades.
With a hard-wall design, like that of the CFM56-7B, the containment wall must be strong enough to prevent the blade from penetrating it. That means the cowling doesn’t have to be as large, so it can have a more streamlined design. But its disadvantage is that the initial broken blade can’t get out of the flow path completely, so it typically stays in the engine as the engine winds down, hitting the other blades.
Some soft-wall designs also have a metal interior wall that blunts the fan-blade projectile as it passes through. Another factor for how the cases are designed is the type of fan blades: Hard-wall composite designs usually won’t work with solid titanium fan blades, only with hollow titanium or composite blades.
To test a soft-wall containment case, the lab researchers fire the broken fan blade into the inside of the ring. Then, they simulate the vibrations caused by the unbalanced fan as the engine winds down. “As it’s spooling down, it goes through different resonances, and you can get very large vibrations,” Pereira says. As with an unbalanced car tire that vibrates violently at certain speeds and not at others, the engine goes through extreme swings in vibration loads at certain points as it slows.
When the engine is under power, the fan blade tips are spinning at supersonic velocities, so the lab researchers fire the broken fan blade into the containment wall at a velocity of about 300 meters per second, Pereira says.
To control the position of the projectile as it leaves the gun barrel, the lab researchers support it in a sabot — a device resembling an aluminum can that fits snugly into the gun barrel and slides through it when the gun is fired, stopping short at the end of the barrel as the projectile flies out. The projectile is typically positioned and supported in the sabot with foam. The researchers fire the gun by releasing pressurized helium or nitrogen into it through a burst valve — a layer of mylar that breaks when heated by a wire, releasing the pressurized gas all at once.
The researchers must then attach the damaged ring to an apparatus on the floor of their lab to simulate the vibrational loads of a blade-out. The damaged ring is bolted flat to a cross-shaped support that has a post in the middle. Four hydraulic actuators extend from the post to connect to the ring at evenly spaced locations Each actuator pushes or pulls with up to 11,000 kilograms of force.
The hydraulic actuators generate the same shear and orbital loading and displacement on the ring as in a blade-out, but not at the same frequency, Pereira says. The hydraulic actuators take 1 second to accomplish what the engine in wind-down mode would experience in a half a millisecond, so it takes several hours to simulate a 30-second wind down.
The containment case passes the vibrational load test if no new cracks form or grow from the initial blade penetration damage. “That kind of loading, if the case isn’t designed properly, it can generate cracks that propagate around the whole engine case,” Pereira says. “If that happened, that would allow the part of the engine to separate, and we can’t have that.”
The FAA requires that no part of the detached fan blade penetrate the outer wall of the cowling in a blade-out, nor can blades that may break off the turbine or compressor parts of the engine, Pereira says. Farther into the flow path, where the turbine and compressor blades are, the FAA doesn’t require certification testing for blade-outs -— only analysis based on previous test data — because the containment wall is stronger and thicker.
Under the High Energy Dynamic Impact project, the ballistics lab has been testing blunt and sharp projectiles fired into flat plates of materials that someday might be approved for fan cases. The materials could also protect fuselages as the FAA would require in designs for planes with open-rotor engines — jet engines without a cowling covering the propulsion blades. Following the flat-plate tests, the researchers began testing materials that have structural features, such as ribs, or variations in thickness, added to them. So far, the lab has performed 200 to 300 impact tests, Pereira says.
The idea is to build a database from impact tests on materials with increasing structural complexity. The data should improve the accuracy of a NASA computer model, Pereira says. A group of companies have started to exercise the model, finding bugs and examining how they can put it to use. These “alpha users” include Pratt & Whitney and Lockheed Martin’s Sikorsky. As the computer modeling of impacts on materials becomes more accurate and capable of predicting the performance of materials, it will help both designers and regulators.
“It’s surprisingly difficult to predict penetration, even in simple metals. If you were trying to predict if a metal projectile will penetrate a flat panel of aluminum, your prediction is probably not going to be accurate.”
Mike Pereira, head of the Ballistics Impact Lab at NASA's Glenn Research Center
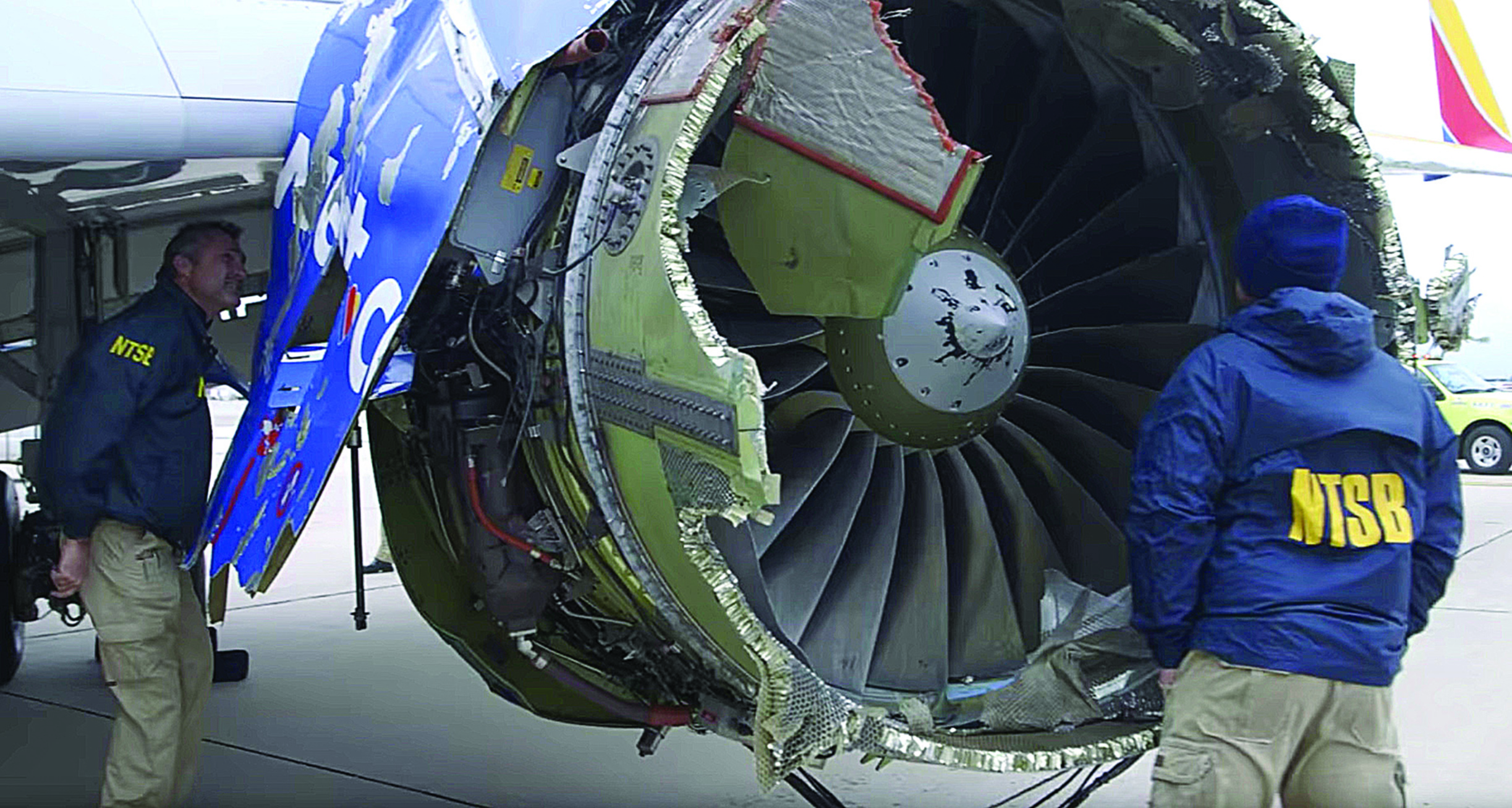