Stay Up to Date
Submit your email address to receive the latest industry and Aerospace America news.
Hardly a day goes by without a space visionary somewhere promising to expand humanity into outer space for good, and billions of dollars are being spent in the belief that this is possible. Among the challenges lies one that is most fundamental: Our human biology cannot survive the radiation for long. Jon Kelvey looks at a possible solution that is struggling for funding.
Elon Musk wants to colonize Mars starting with “a self-sustaining city.” NASA has pledged to return astronauts to the moon, “this time to stay,” though a careful reading shows the agency means by rotating crews to the surface. The China National Space Administration, meanwhile, has said it plans to build a “base” on the moon, though it has not specified whether it would be permanently crewed. For any of this to lead to settlement of the moon or Mars, the harshest reality of outer space must be confronted: It’s full of radiation from our sun and beyond.
Earth’s geomagnetic field, or magnetosphere, protects us from this radiation but gradually weakens as one moves away from Earth, until at the moon, it does a poor job of deflecting radiation. Explorers and settlers there and beyond will face two kinds of radiation: energetic protons that erupt from the sun, and galactic cosmic rays cast off by supernovae. These GCRs consist of protons and heavy ions that race through our solar system in all directions. Without a solution, both forms will ricochet through travelers’ bodies, knocking electrons off of the atoms that make up their cells and DNA. Scientists believe this ionization can not only break DNA, but also harm the ability of cells to repair the damage, leading to an increased risk of cancers, heart disease and cataracts, among other ailments.
In science fiction, writers have taken advantage of forcefield technologies matured in their imaginations to deflect harmful radiation. They recreate Earth’s magnetosphere in miniature so their characters don’t have to live their lives in naturally occurring lava tubes or holes burrowed into the extraterrestrial ground. As it turns out, science fact may not be that far behind. Though, like other radiation researchers, they have struggled for funding, a group of NASA and university scientists have explored the possibility of using “active shielding,” in which large electromagnetic or electrostatic fields would be generated to divert a large percentage of the most harmful radiation away from a surface habitat or spacecraft.
Making such a shield is “a tough problem,” says NASA’s Dan Fry. He is the technical discipline lead in the Space Radiation Analysis Group at Johnson Space Center in Texas and a co-principal investigator for the active shielding research that was begun in 2017 under NASA’s broader RadWorks Project, which also includes modeling and monitoring radiation.
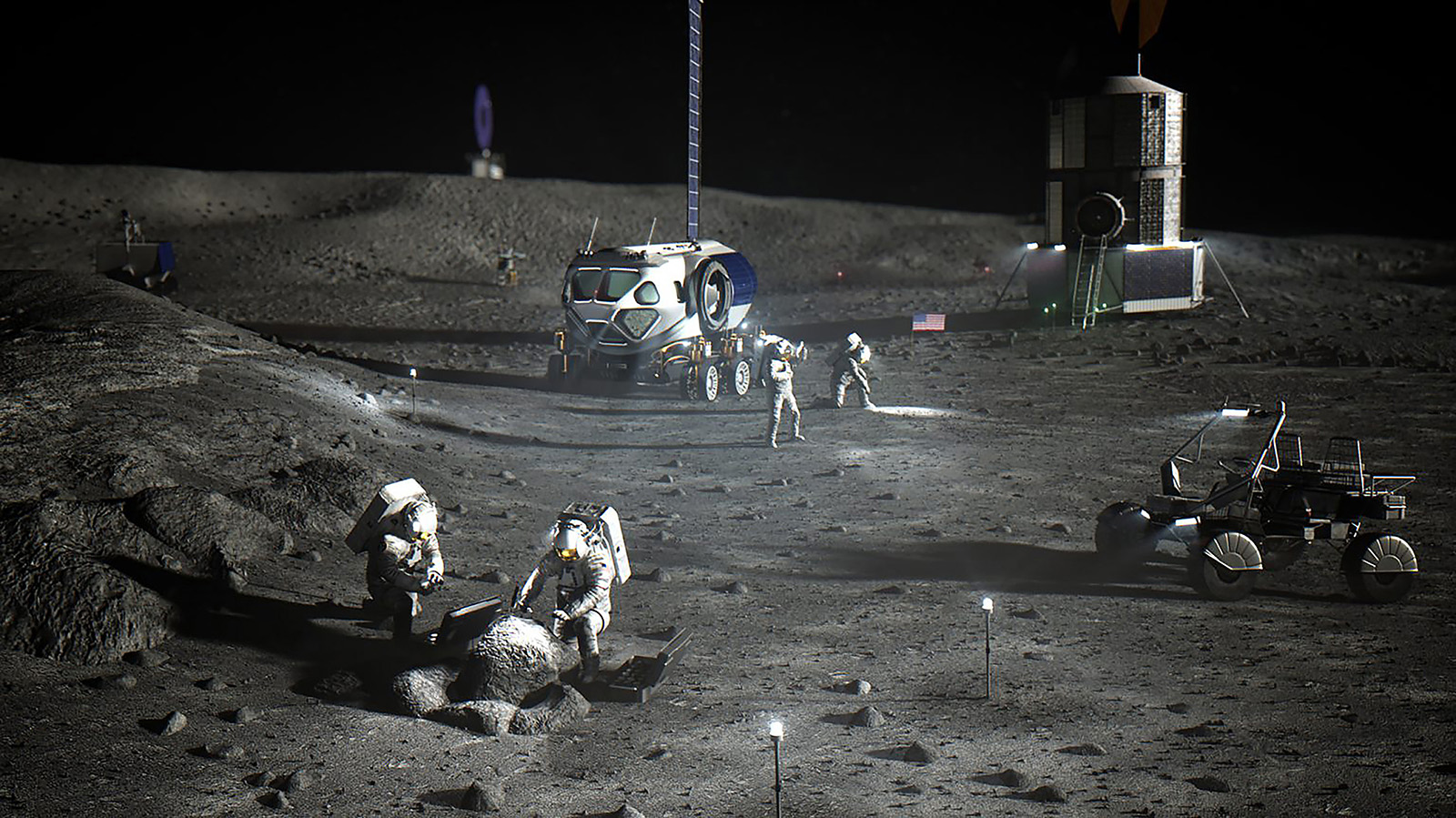
The principles of active shielding are hardly mysterious. In proton therapy centers in the medical field and in particle accelerators operated by physicists, charged particles are regularly manipulated with electric or magnetic fields.
“It’s not a question of ‘We don’t understand the physics,’” Fry says. “The question is,” when you need to make these often heavy, high-powered systems work in space, “‘How do you build it?’”
For four years, Fry and his collaborators studied just that question and made serious, if basic, progress, such as conducting vacuum chamber tests on long, thin field-generating electrodes. They are confident that the good results so far can be maintained once the technology is scaled up to protect full-sized spacecraft and surface habitats.
It’s not that there’s an imminent call for active shielding — none of NASA’s scheduled Artemis missions will last long enough to need it. But NASA has consistently suggested that longer missions are on the drawing board, For example, in 2019, then-NASA administrator Jim Bridenstine said: “This time, when we go to the moon, we will stay.” Several months later, NASA dubbed this plan the “Artemis” lunar program and touted the moon as a “proving ground” for eventual human missions to Mars.
Last year, however, the shielding project was put on hold for reasons that remain unclear. What is known is that NASA needed funding for Artemis III, the first human landing aggressively targeted for late 2025, and funding for active shielding was scaled back to just enough to keep the team together.
“Everybody on this planet has to work within a budget,” Fry says, and right now, the first human landing is “a more pressing priority,” though he cautions that he does not know for certain that his work has been held up for that reason. He hopes that, when the time is right, funding will be restored so the technology can be ready for future Artemis missions.
Space radiation 101
Ionizing radiation consists largely of energetic protons and the fast-flying chips off the nuclei of atoms, known as heavy ions, whether from the sun or outside the solar system as GCRs. Solar protons can range in energy from tens of millions to hundreds of millions of electron volts. In this measure of particle kinetic energy, one electron volt is equal to the energy gained by a single electron accelerated from rest by a 1-volt electric field in a vacuum. And there are a lot of solar protons concentrated in each centimeter of space per second during a radiation storm, a high flux event posing an immediate danger to astronauts, who could develop acute radiation syndrome if caught in such a blast.
But “the protons coming from the sun are low enough energy that you can stop those in a few inches, maybe a foot of material,” says Kerry Lee, the radiation systems manager for NASA’s Orion program.
Each Orion spacecraft contains stowage bays beneath the four crew seats that can be emptied in the event of such a storm. The crew would crawl into the stowage areas to be closer to the craft’s heat shield, and pile the supplies on top of themselves for added protection.
NASA is working with SpaceX on creating a similar shelter aboard the Human Landing System spacecraft that will rendezvous with an Orion in lunar orbit and carry two of the Artemis III astronauts to the surface. NASA in 2021 awarded SpaceX a $2.9 billion contract to build a lunar lander variant of its Starship spacecraft, which will also serve as the astronauts’ habitat during their week on the surface.
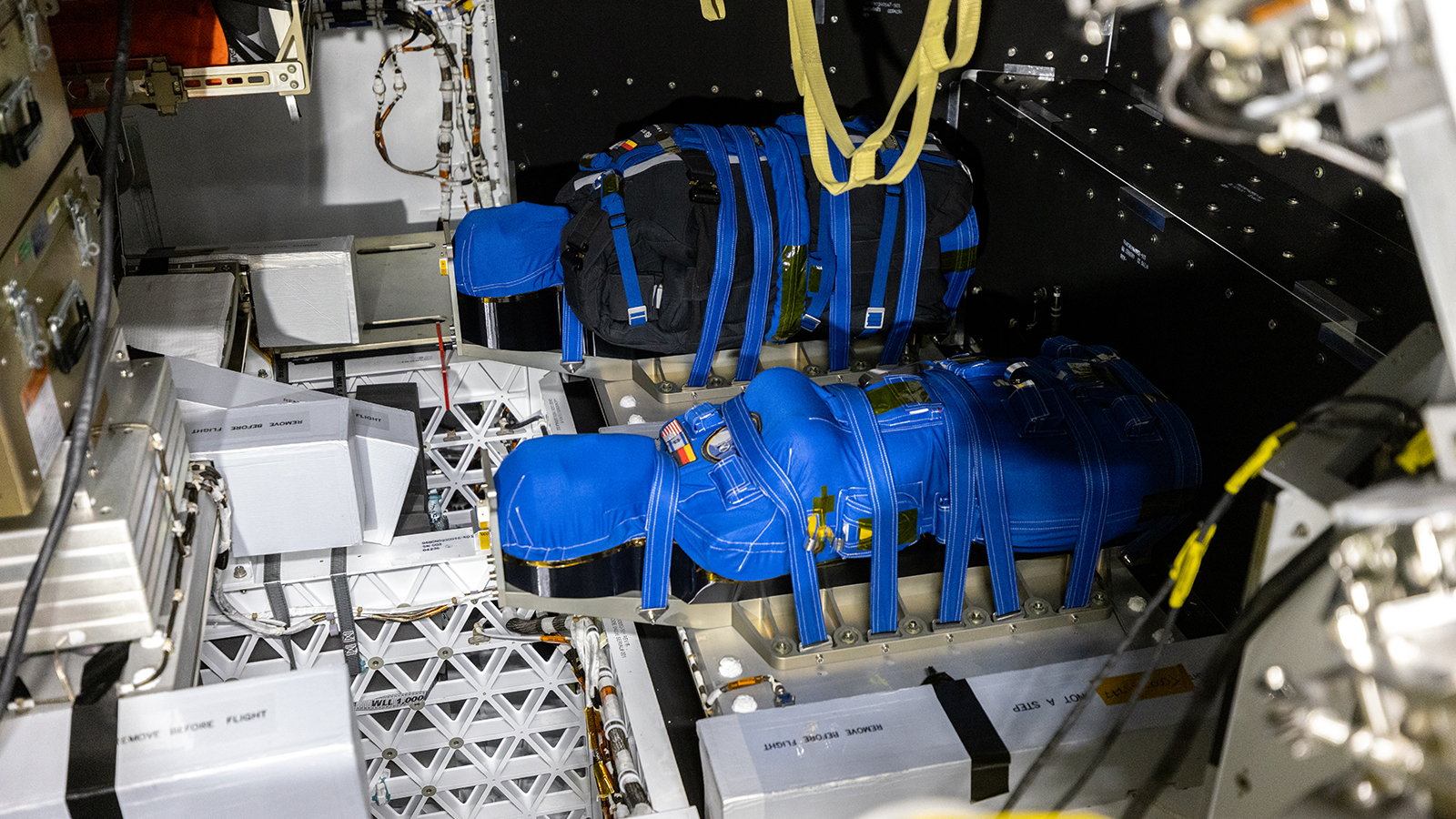
But GCRs are a different beast than solar protons. Though their flux is low, their stream is constant, and their kinetic energy can range from hundreds of millions of electron volts into the trillion-electron-volt range.
“The average or the mean energy of a galactic cosmic ray proton can pass through a meter of material,” Lee says. “You’re not going to fly that.”
It might be possible to move and shape masses of regolith to help block GCRs, he says, but that will require the long-term buildup of infrastructure and the ability to transport massive machinery necessary to conduct such operations on the moon or Mars. And equipment or habitats would need to be designed to be buried.
Other researchers have suggested astronauts find and use caves or lava tubes as natural shelters, “and that’s a reasonable thing to do, except that these things may not be in the area we are interested in going,” Lee says. The early Artemis landings will be concentrated around the south pole, and lunar habitats are currently being designed with landing in the open in mind, “not next to some large rock or some large mountain” that could prove a navigation hazard on descent and landing.
GCRs represent a low enough dose of radiation at any given time that they won’t be a concern for the early Artemis missions, according to Lee, the longest of which is scheduled to last barely more than a month. But when you start looking at deep space missions longer than a year in duration, such as a 24-month roundtrip mission to Mars, that constant background radiation exposure starts to add up.
And that’s where active shielding may come in as an alternative — if, as Fry says, they can figure out how to build it.
The long history of radiation shields
“Active shielding as a potential method to protect the astronauts is not a new concept,” Fry says.
Scientists published on the concept in the mid-1960s, and by 1969, NASA’s Wernher von Braun, the former Nazi who became the agency’s leading space visionary, touted deflecting radiation with electromagnetic fields or plasma fields. Placing superconducting electromagnets, which are coils of wire chilled to cryogenic temperatures to avoid electrical resistance, around a spacecraft could generate a powerful magnetic field. This would be a surrogate for Earth’s magnetosphere, deflecting incoming charged particles so they would miss the core of a spacecraft or habitat, including the crew. A better option, though, due to the size of the components, could be an electrostatic shield that achieves the same result, but by creating a high-voltage electric field. Electrode arrays around a spacecraft charged with a highly positive voltage would exert a repelling force on incoming, positively charged GCR protons. The Soviet Union tested such a shield, in miniature, on the Cosmos 603 satellite launched in 1973 with some success.
But whenever engineers studied putting either concept into action, the required system was too large to be flown in space to protect a full-sized spacecraft or habitat.
“It’s too massive and requires too much power,” Fry says. Let’s say you’re trying to protect the astronauts from a 2-giga-electron volt proton. “You need a lot of power because the field has to be very large.”
Past research efforts showed that 50 to 100 million volts would be needed. So right at the outset of the Active Shielding Project, Fry and his colleagues reevaluated their goals to see if there might be alternative avenues that didn’t lead to such massive, power-hungry systems. They decided to focus on electrostatic shielding because electromagnets are massive and difficult to keep cold enough to be superconducting, whereas it’s relatively easy to place high voltage on an electrode.
In computer models and on a test article in a vacuum chamber at Brookhaven National Laboratory in New York, they tested various configurations, including an array of charged spheres and arrays of positively charged planes with negatively charged rods between them.
“But this configuration is probably not in any way final and would change through further optimization of the problem,” Fry says.
Whatever the optimal geometry, the electrodes surrounding the spacecraft or habitat generate an electric field that diverts incoming GCRs.
They also decided that aiming to divert 100% of GCRs wasn’t worth the difficulty. Given that GCRs represent a low dose of radiation over time, “could we tailor this problem to reducing the dose not to zero, but to get an increase in the number of safe days in space?” Fry says. If they could cut GCR flux by 20%, 30% or 40%, then “you’re making a good dent in it.”
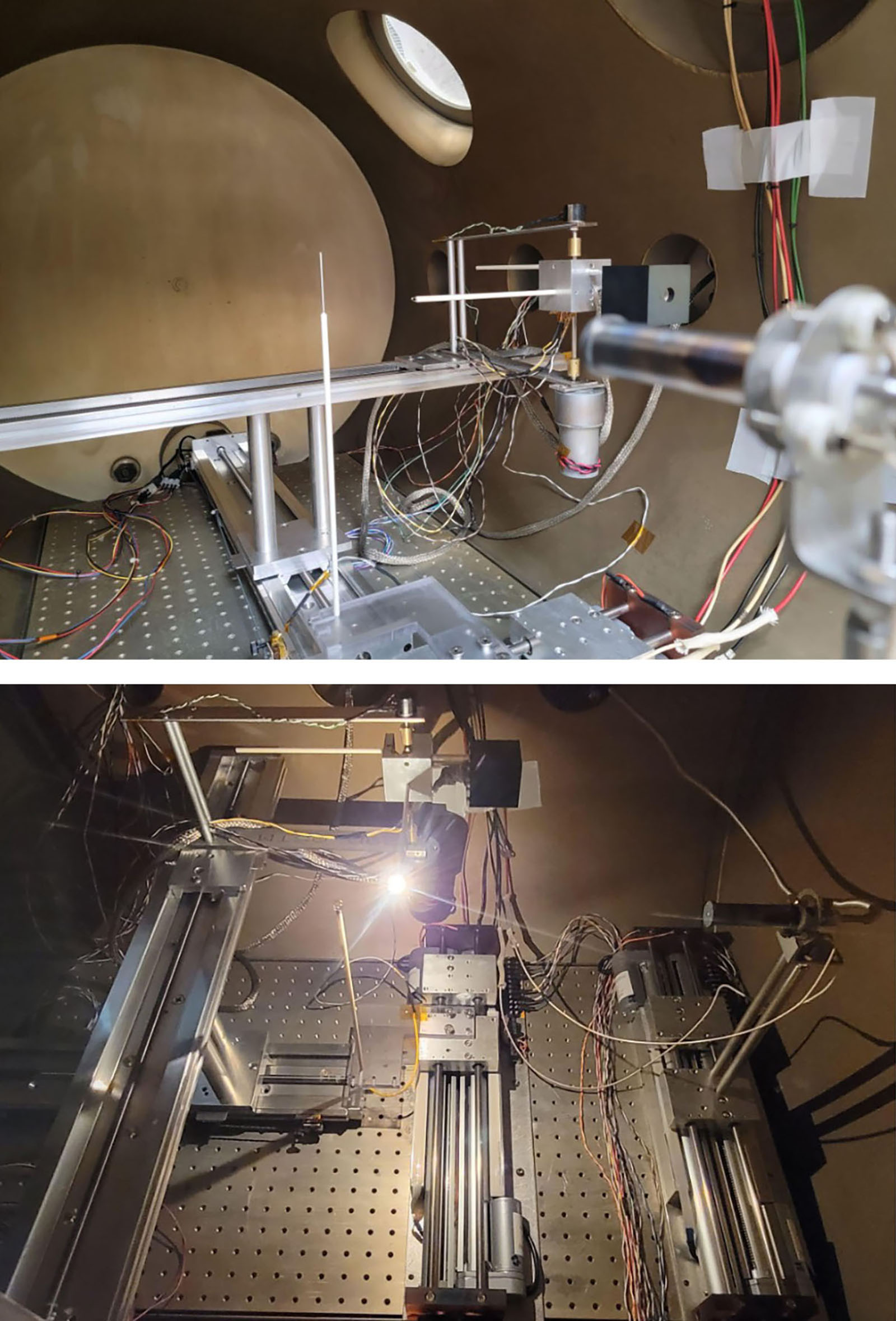
Still, even with a more realistic goal and no electromagnets, electrostatic shielding would require a lot of power — high voltage combined with high current. That’s when Fry reached out to the Space Physics Research Laboratory at the University of Michigan.
“SPRL has been building high-voltage systems to go into space for some time, most notably PIXL on Mars right now,” says Patrick McNally, SPRL managing director.
PIXL, the Planetary Instrument for X-ray Lithochemistry, generates 30,000 volts to power an X-ray beam in a spectrometer with which NASA’s Perseverance rover identifies chemicals in Martian rocks. But an effective electrostatic shield will need a lot more voltage than that — at least hundreds of thousands if not millions of volts. That will require building up charge through multiple stages.
“The first stage might be what’s called the Cockcroft-Walton Voltage multiplier,” McNally says, in which a ladder-like circuit of diode and capacitor stages would step up the voltage from 220 volts to 200,000 volts. A voltage accumulator, such as a Van de Graaff generator, would come next, he says, mechanically moving charge either to conductors to directly charge the shield’s electrodes, or the more “ambitious” idea of using a particle beam accelerator to further boost the charge and transmit it to the electrodes.
The challenge, McNally says, is figuring out just how often you need to charge up your shield. The systems they’ve explored would use positively charged electrodes to deflect positively charged GCR ions, but that will also attract electrons from the charged solar wind plasma that permeates space. That requires a higher current to replenish the charge and maintain the electrostatic field, according to Brian Gilchrist, a professor of electrical engineering at the University of Michigan and director of SPRL.
“It’s easy to charge something up to a very, very high potential if there’s hardly any current,” he says. “If you have to collect a lot of current, it means it takes a much bigger power supply.”
Moreover, “your spacecraft as a whole has several different conductors all over the place. And the sum of all the currents in this entire system always has to be equal to zero,” says Omar Leon, a University of Michigan assistant research scientist who worked on the RadWorks project. “So if you’re collecting a lot of electrons to one conductor, somewhere else in the system, you either have to emit an equal amount of electrons at the same time or collect enough ion current to balance that electron current out.”
The remaining challenges notwithstanding, several findings were made, according to Fry. First, they determined that with a charge of one megavolt, they could reduce solar proton exposure to a crew by about 50%. GCRs remained a tougher challenge, but Fry estimates they could reduce GCR exposure by 25% with a 5-megavolt system — more than can be generated in space at the moment, but much more feasible than a 50- or 100-megavolt system.
Also of importance was the expected mass of the equipment. Fry declined to give the exact mass estimate the team arrived at, but says “our masses were an order of magnitude less than the mass of the vehicle,” referring to a hypothetical spacecraft around the same mass as Orion. Whether intended to shield a spacecraft en route to Mars or a habitat like those that comprise Musk’s vision of a Martian city, a system that’s too heavy just won’t fly.
“We think it can be lighter. We just have more work to do on that,” Fry says. The key is making the electrode arrays as insubstantial as possible. Making a plane with holes in it or, better yet, a planar array of wires eliminates significant mass while still generating a consistent electric field from the point of view of incoming GCRs.
Perhaps most importantly, they discovered dimensionless scaling factors that allow for testing small models in a vacuum chamber and making measurements that will hold even as the model is scaled up to a full-sized craft or habitat, similar to the way subscale aircraft models are tested in wind tunnels.
“That was completely new,” Fry says. “No one had tried developing any type of dimensionless scaling factor in the past.”
Shields (currently) down
If indeed funding picks back up for active shielding, there are aspects of the project that would have implications outside of radiation shielding. Solar sails, for instance, will also generate ionic currents, according to Fry, and so some of the shield research may apply there as well. “You’re in a better financial posture if you can invest in things that are multifaceted,” he says.
At the University of Michigan, the SPRL research is also continuing with limited funding, according to McNally.
“The next step that we’ve been trying to get to is a flight demonstration,” he says. “We know we can learn a lot from ground-based measurements, but you’ve got limitations in a vacuum chamber.”
Fry would ultimately like to try a technology demonstration on the lunar surface. “It could be protecting nothing more than a lander,” he says. “Something that’s like one cubic meter in size.”
In the meantime, the project has accomplished fundamental research on active shielding that McNally likens to what early aircraft engineers had to go through to achieve stability in the air.
“They started off understanding lift and drag” before understanding stability, he says, and that led to wind tunnel testing to come up with stability derivatives and the lift-drag curves essential to actually engineering an airplane. “All of that kind of information was very empirical in the beginning.”

About jon kelvey
Jon previously covered space for The Independent in the U.K. His work has appeared in Air and Space Smithsonian, Slate and the Washington Post. He is based in Maryland.
Related Posts
Stay Up to Date
Submit your email address to receive the latest industry and Aerospace America news.
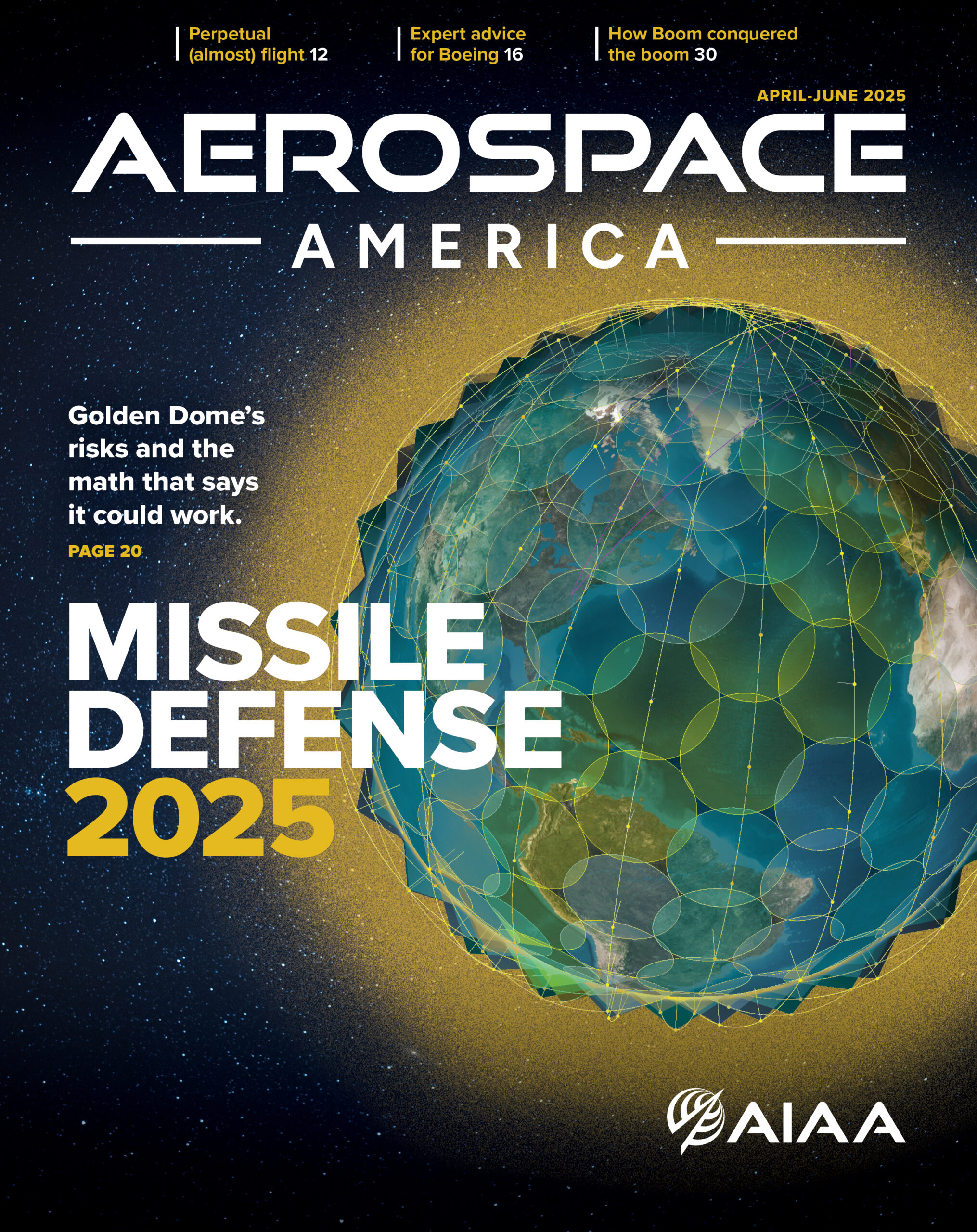