Going broadband
September 2017
Airline passengers and crews can look for some bold innovations in technology
The number of airliners equipped for broadband connectivity from ground stations and satellites could nearly quadruple to 24,400 by 2026, estimates Florent Rizzo of Euroconsult, a space-market consultancy with offices in France, the United States, Canada and Japan.
Business passengers want to stay productive in flight, and leisure passengers want to be entertained and to remain in touch. Broadband also gives the crew, with cyber-security in mind, better weather data and bigger data pipes for downloading operational and maintenance data. All customers want more bandwidth and less expensively.
The question is how best to provide that bandwidth. For satellite connectivity, communications companies are vying to improve the equipment required on the aircraft. A move is underway to replace heavy, drag-inducing mechanically steered antennas with electronically steered antennas, or ESAs, which are on the horizon. There may even be a way of shedding the need for satellite connectivity altogether. Why not use the massive fleets of commercial aircraft themselves as the link in a giant mesh network of broadband connectivity for both aircraft and other users?
Here is a rundown of the technical choices:
Better antennas
Aircraft connectivity started in the United States with ground stations linking to small antennas on the bottom of fuselages. As aircraft began to fly over oceans and areas with less ground infrastructure, satellites links became necessary. For broadband, these have generally been satellites in geosynchronous equatorial orbits 35,000 kilometers over the equator launched by firms like SES or ViaSat. Aircraft link to these satellites with dish antennas that mechanically change directions to focus on the satellite and maximize reception.
Dish antennas have some downsides. They must be protected against airflow with radomes, 21 or more centimeters in height. Radomes add drag, especially on takeoffs and landings. The mechanically steered antennas inside add weight. Including structural strengthening, radome and the antenna itself, this option typically weighs about 182 kilograms (400 pounds), according to David Brunner, global sales vice president of Panasonic Avionics. Panasonic and similar firms assemble connectivity equipment and services and offer the package to airlines.
ESAs could eventually be a much better choice. These are software-controlled, phased-array antennas whose beams are electronically steered in different directions without moving the antenna. They can lie flat, conforming to the shape of the fuselage, eliminating drag. ESAs can be light and, with no moving parts, much less prone to breakdown.
Aircraft ESAs have been made for military uses, but expensively, up to $10 million. More affordable ESAs have been developed for the L band, from 1 to 2 gigahertz, in 2003 and are now available for S band, from 2 to 4 GHz. But these are relatively narrow bands sufficient for limited messaging by pilots and perhaps a few passengers, not well suited for the massive volumes of data desired on commercial aircraft.
Broadband connections must work in the much more spacious Ku or Ka bands, from 12 to 18 GHz or 26.5 to 40 GHz, respectively. Here the challenges include maintaining connections at high latitudes, limiting power requirements and ensuring performance under extreme temperatures, all while getting costs down.
David Helfgott, CEO of Arlington, Virginia-based Phasor, believes he is nearing the goal of an effective broadband ESA for commercial aircraft. “We have been working hard for years, we are way ahead and well-positioned,” Helfgott says.
Making even conventional antennas for Ku and Ka bands is tougher than for the narrower S and L bands because regulations set much stricter rules on how beams must behave, can be used and interact with satellites. This usually requires a larger antenna, heavier and with a taller radome.
Supporting broadband with phased-array ESAs is also hard. Phasor combines microchips with a network of omni-directional patch antennas, 5 millimeters by 5 millimeters, which are the radiating elements. These elements combine and steer communications electronically. About 500 elements are grouped in a module.
An aircraft ESA might have six, 12 or more of these modules. The modules would be 6.4 centimeters thick, with covers, versus 21 or more centimeters for radomes. A six-module Phasor ESA weighs less than 12 kilograms.
No moving parts should mean much less maintenance. For example, United Kingdom-based Cobham expects to get 100,000 hours mean time between removals from its L band ESAs, versus 20,000 hours for its mechanical models. Helfgott of Phasor says his ESA should be much more reliable than traditional antennas.
Perfectly flat ESAs may lose connectivity at latitudes of 55 to 58 degrees because their look angle to geosynchronous satellites over the equator is blocked by the horizon. Helfgott says his conformal ESAs, which wrap around the curve of the fuselage top, will maintain connections farther north, but acknowledges they will eventually lose connection as the poles are approached. He expects that widely dispersed constellations of low Earth orbit satellites in the near future, such as those planned by OneWeb, Telesat, LeoSat, Kepler and perhaps SpaceX, will eliminate this problem.
He says the Phasor ESA’s ability to send and receive two beams on a single aperture will enable it to manage two links simultaneously, essential to manage handoffs smoothly among low Earth orbiters.
Active phased arrays require power, and though each chip needs only micro-current, the entire array requires much more. Helfgott says his ESA has constrained the power required to well below that available on commercial aircraft, and has ensured that dissipating the heat from this power will not be an issue.
Aircraft antennas must work over a wide range of ambient temperatures, from as low as minus 70 degrees Celsius in the air to as high as 90 degrees Celsius on desert tarmacs. Helfgott says active phased arrays will deal with cold temperatures and Phasor has solved the desert problem.
On cost, Helfgott says his ESA was designed from the beginning for efficient manufacture and will use global supply chains for both chips and printed circuit boards. Panasonic’s Brunner notes that making ESAs for an addressable market of tens of thousands of aircraft should also enable scale economies. Helfgott says he will be able to offer efficient, flexible ESAs at prices comparable to the best mechanical models.
The Ku-band version of Phasor’s ESA antenna has completed Technology Readiness Level 7, having demonstrated a prototype in an operational environment. Beta testing for TRL 8, demonstration and certification of the actual system, will begin in the third quarter of 2017. This certification step could take six to 18 months, and Helfgott hopes to offer this first aircraft ESA to the market in the second half of 2018.
Other firms are also active. Redmond, Washington-based Kymeta is developing flat, light broadband antennas to connect satellites to mobile platforms, including ships, trains, buses, cars and aircraft. It is working with firms that supply inflight connectivity to commercial aircraft.
Kymeta’s approach differs from the phased arrays used by Phasor, stresses Steve Sybeldon, vice president for business development. Kymeta uses metamaterials, artificially engineered materials similar to those used in liquid crystal display television sets. The tunable metamaterial elements create a holographic beam that transmits and receives signals, and software points the beam. This technology does not require active phase shifters, so it requires very little power.
Kymeta products have met environmental requirements for maritime operations, but aviation will require even lower temperatures. The firm is working with suppliers to modify its components for certification on aircraft. Trials and evaluations are underway for the aircraft version of Kymeta’s product, which it calls the mTenna.
Euroconsult’s Rizzo expects phased-array ESAs to enter the market in 2018, including one made by QEST, based in Holzgerlingen, Germany. He predicts Rockwell Collins and Gilat Satellite Networks, based in Gilat, Israel, will also offer ESAs in coming years. NLR, the Netherlands Aerospace Center, has been working with private partners on ESAs. As always with commercial aircraft, certification must follow innovation to yield practical application.
Brunner is eager for effective ESAs but cautious on timing. He judges practical and economic ESAs won’t be ready for 24 to 48 months. In the meantime, Panasonic might install hybrid ESAs, which are thinner and lighter than today’s equipment and change direction partly mechanically and partly electronically.
A mesh network of aircraft?
Even the best ESAs need satellite links, so connectivity must cover the cost of satellites and their launches. A new high-throughput satellite — which provides two to 20 times the broadband capacity of older satellites — costs about $250 million, and launching it can run from $60 million to $130 million, according to Euroconsult’s Rizzo. Each month, an airline must pay about $3,400 for each megabit per second of capacity from older satellites or about $600 per megabit per second provided by a high throughput satellite. That price difference is one reason more airlines are adopting broadband.
Rizzo expects satellite connectivity costs to decrease another 30 percent in the next three years due to next-generation satellites. Even so, with tens of thousands of passengers downloading files or streaming movies each day, those capacity charges mount up to serious money.
Enter Airborne Wireless Network, a startup based in Simi Valley, California. To reduce the need for aircraft to communicate via satellite, it wants to develop a mesh network of aircraft that would relay broadband signals among them and to other users. The network would link to ground stations wherever it chose based on least-cost considerations.
“The more ground stations we have, the more we can reuse spectrum and the more capacity we will have,” explains Marius de Mos, vice president of technical affairs and development.
For the U.S., with about 5,500 aircraft flying, that would eventually mean about 200 ground stations, but Airborne could start with much fewer. It might begin by equipping several airlines on designated corridors and building a couple of dozen ground stations, de Mos says.
Aircraft would need radios, modems and antennas to repeat signals. The system would supply broadband to aircraft, but could also serve other users, especially in rural areas not covered by ground networks. Airborne would not compete with aircraft connectivity providers, but supply them by supplementing satellite or ground connections.
The system can work at 240 nautical miles (444 kilometers) between aircraft, even 600 nm (1,111 km) over oceans. But at full U.S. implementation, the network should average about 60 nm (111 km) between planes. “The more density, the more bandwidth,” de Mos notes.
Outside of densely populated tracks, fewer aircraft fly over oceans, so Airborne might put some repeating equipment on ships.
De Mos says the network would start by supplying megabits per second, then move to 10s of gigabytes, enough for both aircraft and other users on the ground. When fully built out, it could handle terabytes. “No one else could do that,” he stresses.
Airborne initially would mount three to four antennas on top and bottom of a fuselage. These would be low-profile, low-drag hybrid ESAs in radomes about 15 centimeters high, until full ESAs are available. Eventually, full ESAs would be used.
Radios and modems would be standard equipment, customized for Airborne’s purposes. One advantage is that these devices could be upgraded quickly by just swapping boxes, as technology improves. Satellites, in contrast, are designed two years before launch and then operate for five to 10 years. By definition, old satellite technology stays in use for a long time.
And the mesh approach means that when one link is down or blocked by weather, another can instantly replace it. Each aircraft would have three repeaters. Quick repair of connectivity is not possible with current satellites.
In May 2017, Airborne completed a proof of concept using two Boeing 767s for a ground-to-air-to-air-to-ground link. Next in 2018 will come a test on 20 aircraft emulating a global network. This test will use production-ready equipment. De Mos expects rollout in 2019.
De Mos acknowledges he needs a critical mass of aircraft to make the approach work. He is talking to Air Lease, which leases several hundred aircraft to airlines and has ordered several hundred more. He expects airlines to line up because they want much more reliable connections for passengers, and Airborne’s mesh approach would support that. Also, by supplying connectivity to other users, airlines might convert a pure cost burden into a revenue earner.
“The science is there, and they are smart guys,” says Brunner. “The problem is how to penetrate a critical mass of aircraft.”
Broad adoption seems to be a condition to make sure aircraft stay connected in the mesh network, Rizzo observes. Still, “the solution could be a complementary service to provide connectivity.”
In any case, several paths to better aircraft broadband are opening up. It’s a tricky but happy dilemma for airline managers.
A new high-throughput satellite — which provides two to 20 times the broadband capacity of older satellites — costs about $250 million, and launching it can run from $60 million to $130 million.
Florent Rizzo of Euroconsult, a space-market consultancy
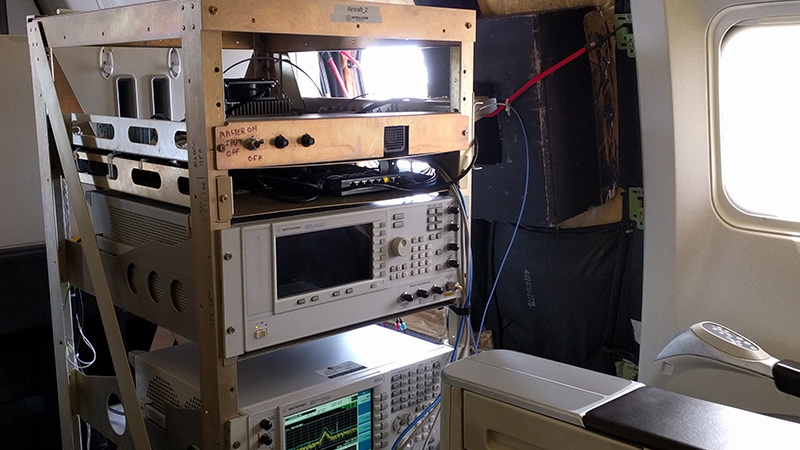
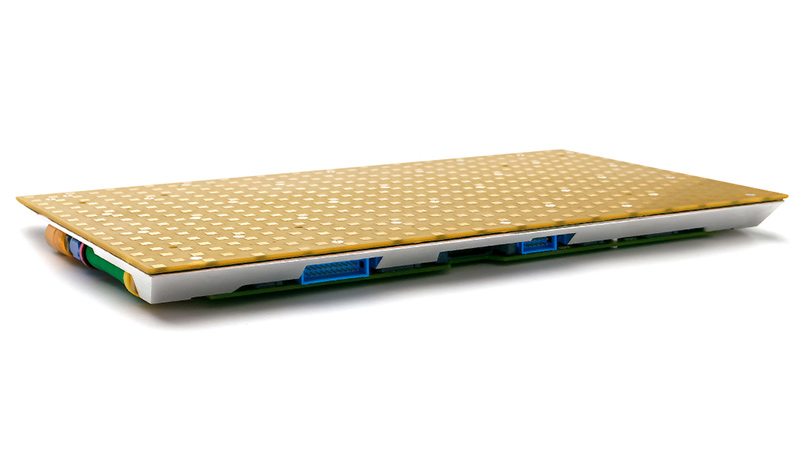