- AIAA Announces 2023 Sustained Service Award Winners
- Making an Impact: Students Present Research at Annual YPSE Conference
- Don’t Miss “Teacher Friday” on 27 January
- University of Adelaide Hosts 2022 AIAA Region VII Student Conference
- SAT OC’s Successful Year
- AIAA Standards Under Revision
- Integrating Mission Objectives, Capabilities, and Technologies — 2022 SSTC Essay Contest Winners Announced
- Describe a Space Mission Designed to Achieve a Specific Objectives (1st Place, 8th Grade)
- Describe a Space Mission Designed to Achieve a Specific Objectives (2nd Place, 8th Grade)
- Describe a Space Mission Designed to Achieve a Specific Objectives (3rd Place, 8th Grade)
- Describe a Space Mission Designed to Achieve a Specific Objectives (1st place, 7th Grade)
- Describe a Space Mission Designed to Achieve a Specific Objectives (2nd place, 7th Grade)
- Describe a Space Mission Designed to Achieve a Specific Objectives (3rd place, 7th Grade)
- AIAA Fellow Gasich Died in January 2022
- AIAA Fellow Boehm Died in August
- AIAA Associate Fellow Tauber Died in October
- AIAA Fellow Mueller Died in December
Bulletin
AIAA Announcements AIAA Announces 2023 Sustained Service Award Winners
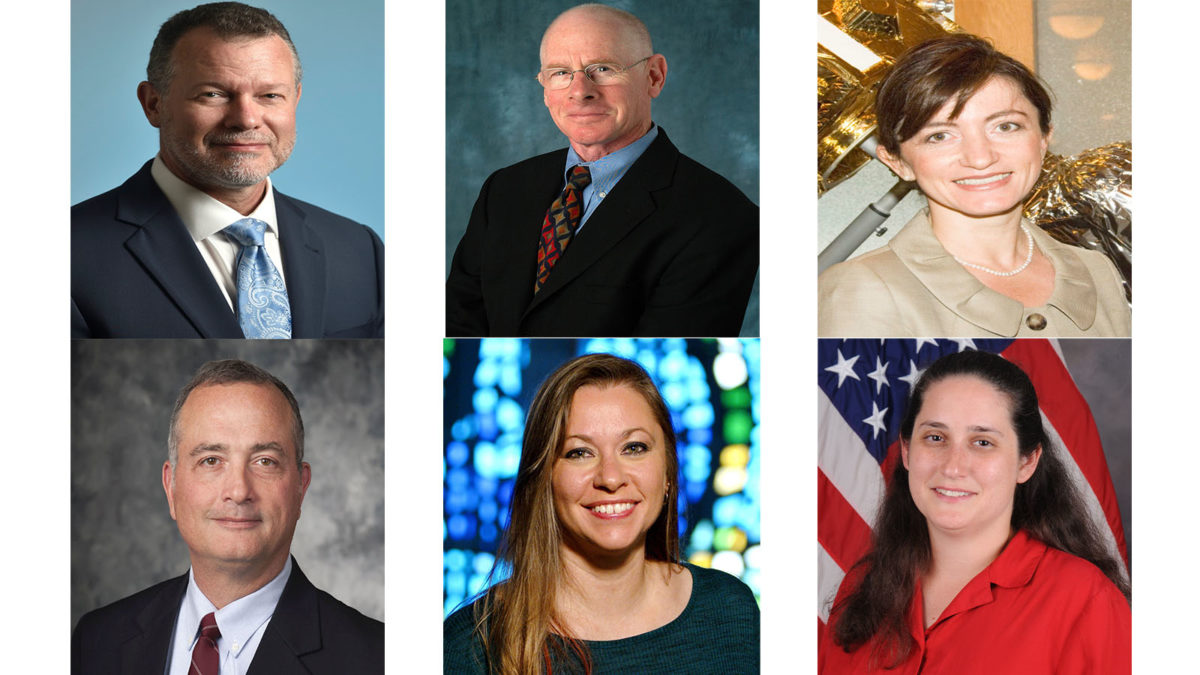
AIAA has announced the winners of the 2023 Sustained Service Awards. The award recognizes “sustained, significant service and contributions to AIAA by members of the Institute.” Recipients must be AIAA members in good standing who have shown continuing dedication to the interests of the Institute by making significant and sustained contributions over a period of time, typically 10 years or more. Active participation and service at the local section/regional level, and/or the national level is a potential discriminator in the evaluation of candidates.
The 2023 Sustained Service Award winners are:
David L. Carroll
CU Aerospace LLC
For distinguished and sustained service to AIAA; the enhancement of science, innovation, and entrepreneurial leadership in aerospace engineering; and education of scientist engineers.
Carroll co-founded CU Aerospace in 1998 and has served as its president since 2011. The company’s focus is engineering innovation for new aerospace technology products. He received his Ph.D. in Aerospace Engineering from the University of Illinois in 1992. He was inducted as an AIAA Fellow in 2011.
John W. Daily
University of Colorado Boulder
In recognition of sustained contributions to the Institute’s technical services, publications, and education committees.
Daily is Emeritus Professor of Mechanical Engineering at the University of Colorado Boulder. He received his Ph.D. from Stanford University in 1975. He works in the field of combustion and fire. He has served AIAA in numerous positions and is a Fellow of the Institute.
Luisella Giulicchi
European Space Agency
For over two decades of service to the Institute’s governance, regional organization, and technical activities, and for being the advocate of international cooperation and AIAA engagement worldwide.
Giulicchi is a system manager at the European Space Agency (ESA), The Netherlands, for the Copernicus Program: the largest operational Earth observation program in the world. Her technical and programmatic management contributions include Bepi Colombo, SMART-1, LISA Pathfinder, Copernicus Sentinel-1, and Copernicus Sentinel-6. She is an RAeS Fellow; AIAA Associate Fellow, WIA-Europe President, and past AIAA Board of Directors member.
Walter O. Gordon
Moog Inc. (retired); Colonel, U.S. Air Force (retired)
For exceptional service to AIAA and for significant advancement of aerospace activities.
Gordon worked as an engineer in Western New York for 41 years before retiring recently to devote his time to the AIAA Niagara Frontier Section and local aerospace history. He also flew C130s in the Air Force Reserve, retiring in 2014 as the commander of the 914th Airlift Wing.
Dawn Phillips
NASA Marshall Space Flight Center
In honor of 20 years of dedicated service, leadership, and tireless work on behalf of AIAA, the AIAA Structures Technical Committee, and AIAA members.
With NASA, Phillips has supported the Space Shuttle, Ares, and SLS programs as a stress analyst and member of the NESC Structures Team. She is currently in Huntsville, AL, as the MSFC Assistant Chief Engineer for the International Space Station.
Sidra Silton
U.S. Army DEVCOM Army Research Laboratory
For two decades of dedicated service to the applied aerodynamics and fluid dynamics technical communities.
Silton received her Ph.D. in Aerospace Engineering from the University of Texas at Austin in 2001. Upon graduation she began working for the Army Research Laboratory where she now serves as Chief of the Mechanical Sciences Division. She is an AIAA Associate Fellow.
Section News Making an Impact: Students Present Research at Annual YPSE Conference
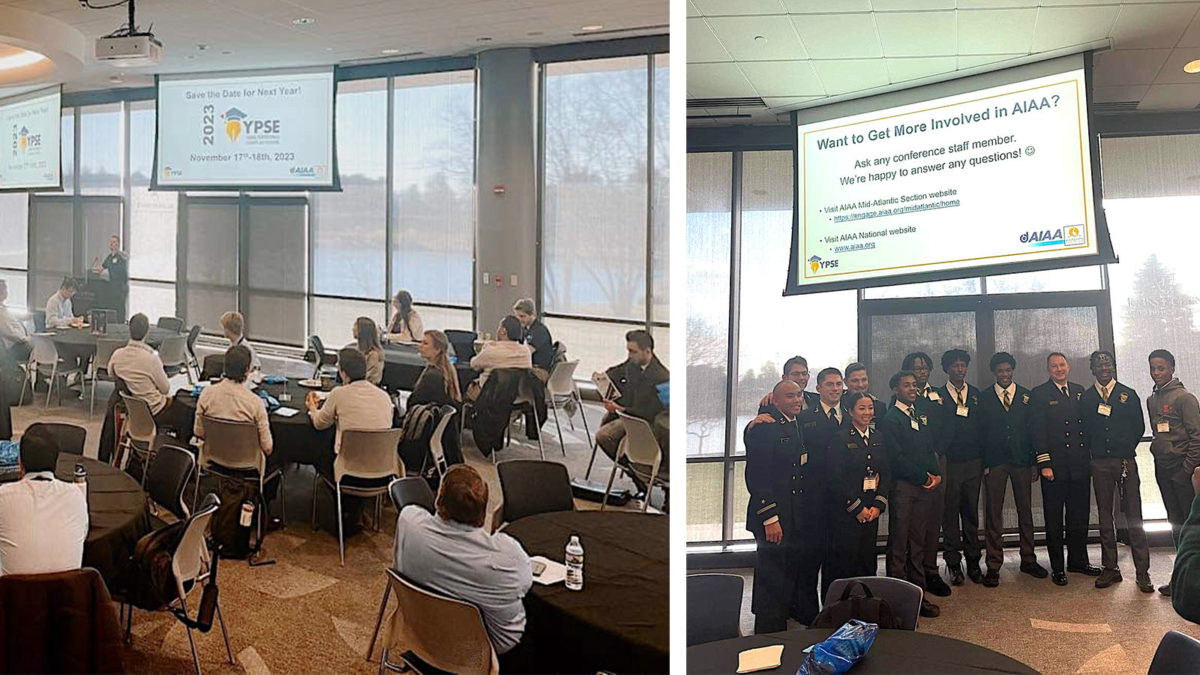
The AIAA Mid-Atlantic Section held their annual Young Professionals, Students, and Educators (YPSE) Conference in November. University and high school students presented their research at the event, with the addition of young professionals and educators also in attendance at the Johns Hopkins University Applied Physics Laboratory in Laurel, MD.
The conference was an incredible experience that brought together students, educators, and young professionals from all around the country to share their work and make connections within the broader aerospace industry. In addition to the presentations, the conference provided an opportunity for attendees to network and learn about various aspects of the aerospace industry and academia. AIAA Headquarters also hosted an exhibitor table where staff talked to attendees about upcoming events, programming, and AIAA membership.
The winning presentations included:
High School Category:
Honorable Mention – Logan SmithPerkins, The Effect of High Forces at Launch on the Deformations of a Protected Object
Top Presentation – Khoi Dinh and Alan Hsu, TJREVERB: Novel Communications and Radio Configuration for Educational CubeSat Missions
Undergraduate:
Honorable Mention – Jerry Liu and Kaylyn Song, Finding the Scaling Law for Pulsejet Engines
Top Presentation – Matteo Cerasoli, Ionospheric Propagation Measurement Through the Use of Sounding Rockets
Graduate:
Honorable Mention – Abenezer Taye and Peng Wei, Scalable Real-Time Trajectory Planning Framework for Urban Air Mobility
Top Presentation – Jorge Ahumada Lazo, Characterization of Recirculating Structures in the Near Field of Underexpanded Swirling Jets
Young Professional:
Honorable Mention – Erin Sutton, Preliminary
Use for System Identification to Validate Models of Dragonfly’s Octocopter
Top Presentation – Georgios Kyriakou, Additively Manufacturing Electronics: From Lab to Outer Space
Educator:
Top Presentation – Michelle Ming, Exposure Deficit
AIAA Foundation Don’t Miss “Teacher Friday” on 27 January
Are you a K-12 educator near the DC/Maryland/Virginia area? You are invited to a free professional development workshop taking place at AIAA SciTech Forum!
When: Friday, 27 January 2023, 0800–1630 hrs ET USA
Where: Gaylord National Resort and Convention Center, National Harbor, Maryland
Meet educators and engineers and learn about the aerospace challenges of the 21st century. Dive into STEM concepts for your classroom or afterschool club/organization. Attendees will discover newly developed standards-based curriculum and integrated projects that can be used in the classroom. Topics include:
• Cutting-edge rocketry curriculum introduction (for students of all ages)
• An insider’s look at NASA’s Artemis program
• Aircraft Design Challenges
• DEI considerations for STEM programs
• High school CubeSats
• The value of communication and teamwork in STEM
• The Martian Greenhouse Program
• And more
Register at aiaa.org/scitech/registration. Select one-day registration for Friday, 27 January, at checkout and use code ST23TF for free* registration.
*Please note: This registration code may only be used by K-12 educators.
This event is organized and hosted by the AIAA STEM K–12 Outreach Committee.
AIAA Foundation University of Adelaide Hosts 2022 AIAA Region VII Student Conference
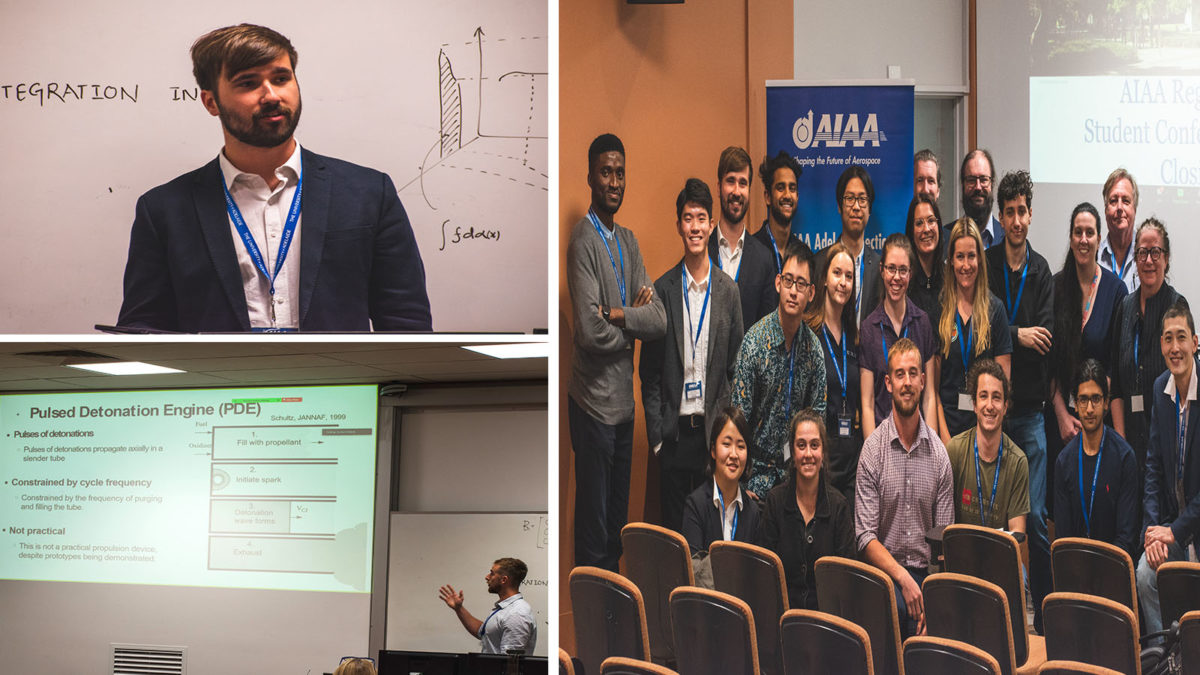
To wrap up the 2022 Regional Student Conferences, the University of Adelaide hosted the 2022 Region VII Student Conference, 29–30 November 2022, both in person and virtually. There were 34 papers presented, representing 23 universities. The conference had a strong international presence with students from 12 countries, including Australia, Bangladesh, China, Germany, India, Italy, Japan, Netherlands, New Zealand, Paraguay, the United Arab Emirates, and the United Kingdom.
Students presented in three categories: High School, Undergraduate, and Masters. Their presentations were evaluated by industry peers with many years of experience in the aerospace sector.
First-place winners in the Undergraduate and Masters categories received a cash prize of $500 and an invitation to participate in the International Student Conference at the 2023 AIAA SciTech Forum, 23–27 January, National Harbor, Maryland. Second-place winners received a cash prize of $300, third-place winner received $250, and the high school winner received $100.
High School Category
ShivNaveed Raina, Scholars International Academy, United Arab Emirates, “Exploring How the Hybridisation of Laser-Microwave Hybrid Wireless Power Transfer System (LMHWPTS) has Increased Efficiency in Comparison to the Two Commonly Established Traditional Models”
Undergraduate Category
1st: Daniel Smith, Monash University, Australia, “Symmetry-Enforced Coherent Structure Background Oriented Schlieren”
2nd: Omar Mourad, University of Stuttgart, Germany, “Neural Network based Model-Predictive Upset Recovery Control in Real-Time”
3rd Tie: Michael Pangestu, Monash University, Australia, “Enhancing 2-Component – 2-Diminsional Particle Image Velocimetry Using Physics-Informed Deep Learning”
3rd Tie: Kevin Liu, Monash University, Australia, “Enhancing Large Eddy Simulation Sub-grid Scale Closure Model Estimation Using Convolutional Neural Networks”
Masters Category
1st: Vishal Kashyap, Queen Mary University of London, United Kingdom, “Reinforcement Learning Based Linear Quadratic Regulator for the Control of a Quadcopter”
2nd: Celine Jane, Victoria University of Wellington, New Zealand, “Magnetic Disturbance Analysis of Spacecraft using Electromagnetic Thrusters”
3rd: Aaron Sew, Royal Melbourne Institute of Technology, Australia, “Designing an Automated Barking Drone to Detect and Repulse Cattle in Real World”
AIAA’s student conferences are a way for students to present their work in front of their peers and members of the industry. The Regional Student Conferences for Regions I-VI take place annually in the spring.
AIAA would like to thank Lockheed Martin for supporting the program. Additionally, special thanks to the University of Adelaide, the Adelaide Section, the judges, Rey Chin, Patrick Neumann, and Region VII Director Cees Bil for coordinating the conference.
AIAA Committees SAT OC’s Successful Year
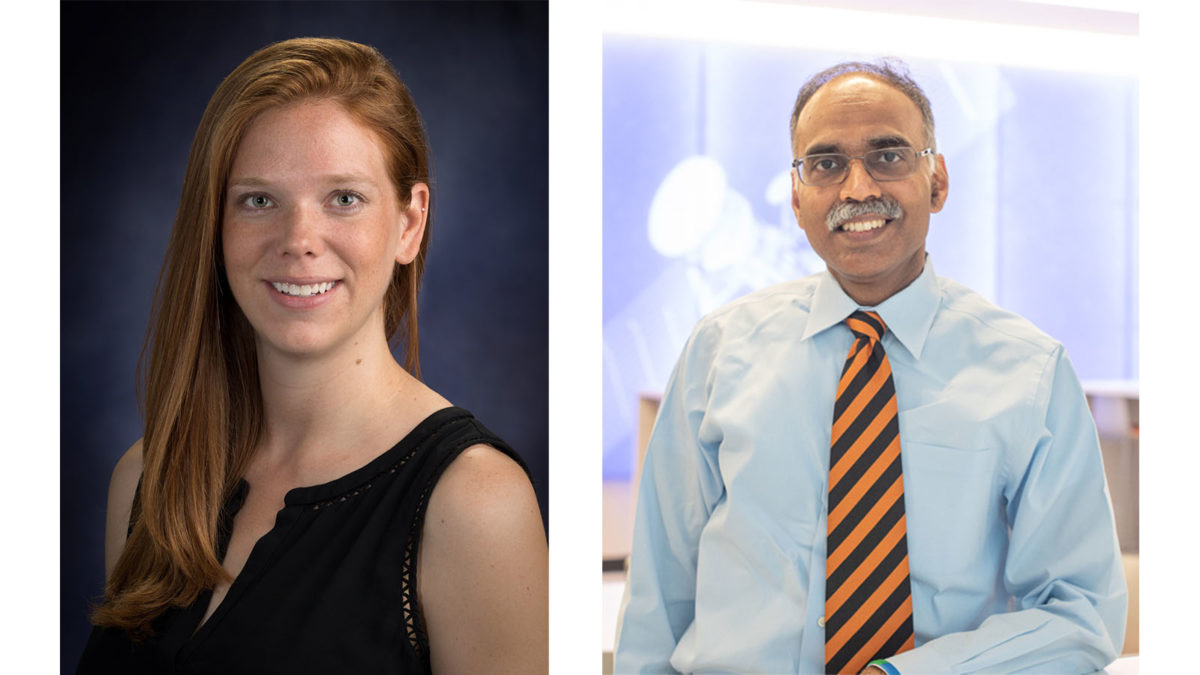
By Amir S. Gohardani, SAT OC Chair
The AIAA Society and Aerospace Technology Outreach Committee (SAT OC) had a very productive year, seeing its largest membership in the past 7 years with a 32% growth of committee members since 2021. Based on the shown interest in the committee and the number of membership applications submitted for 2023, this year will be an even better year with an increasingly diverse membership active in both for-profit and nonprofit organizations, as well as government agencies. The successful launch of the Diversity Corner in collaboration with the AIAA Diversity and Inclusion Working Group, SAT OC also has paved the way for the committee to take new steps in its commitment to become more inclusive. Continuing its tradition of exploring a myriad of topics related to aerospace technology and society, the SAT OC will host three sessions during the 2023 AIAA SciTech Forum. We invite you to join these sessions and assist us in advancing the discussions on society and aerospace technology.
SAT OC Spotlight
This month, we are spotlighting Elizabeth Rieken who joined the committee in April. Rieken is an aerospace engineer in the Engineering Integration Branch at NASA Langley Research Center. She holds a B.S. in Aerospace Engineering from the University of Virginia and an M.S. and Ph.D. in Mechanical Engineering from Stanford University. Rieken conducted graduate research in the field of laser diagnostics prior to completing her dissertation work focused on increasing innovation in engineering design through mindfulness and divergent thinking. Her training and experience span the disciplines of engineering, design, education, and social science. She joined NASA Langley in 2018 as a researcher in the Aerothermodynamics Branch. Her work focused on aeroheating and aerodynamic force and moment wind tunnel testing for hypersonic vehicles. In 2021, Rieken joined the Convergent Aeronautics Solutions (CAS) project as a complex systems design engineer. CAS explores pressing sociotechnical problems and imagines desirable aviation futures to illuminate high-value opportunities for NASA aeronautics and beyond. She leads the Synthesis element of the CAS lifecycle where teams focus on problem exploration and problem framing with a systems-level lens. She is thrilled to exercise the breadth of her training and expertise in aerospace engineering, design, and social science to think differently about framing problems for aviation concepts.
Upon joining SAT OC, she is equally excited to expand her community of students and professionals who conduct and promote work at the intersection of aerospace technology and complex societal challenges.
Diversity Corner
Name: Maruthi Akella
Notable Contributions: Akella holds the Ashley H. Priddey Centennial Professorship, at the University of Texas at Austin and is director of the Center for Autonomous Air Mobility. An expert in space robotics, autonomy, learning, and control theory, Akella was elected to the 2022 AIAA Fellow class “for sustained outstanding scholarship, leadership, and high-impact contributions in the field of aerospace guidance and control.”
Akella is also a Fellow of the IEEE and the American Astronautical Society (AAS) and holds the Academian rank with the International Academy of Astronautics. His major research contributions have been recognized through the AIAA Mechanics and Control of Flight Award (2014); IEEE AESS Judith A. Resnik Space Award (2015); IEEE Control Systems Society Award for Technical Excellence in Aerospace Control (2020); academician, International Academy for Astronautics (2020); and AAS Dirk Brouwer Award (2020). Akella is currently the Editor-in-Chief of The Journal of the Astronautical Sciences and an Associate Editor for the AIAA Journal of Guidance, Control, and Dynamics. He is the Technology area coordinator for the State of Texas Urban Air Mobility Advisory Committee. He also serves on the AIAA Guidance, Navigation, and Control Technical Committee and the AAS Board of Directors.
Potential Societal Impact of Contributions: Along with his many impressive contributions to the aerospace controls field, Akella made many enduring educational contributions as an academic advisor and mentor. With a classroom philosophy of fostering open discussion and embracing ambiguity, he encourages his students to be curious and embrace the lessons learned from both successes and failures.
*In collaboration with the AIAA Diversity and Inclusion Working Group and Claudine Phaire, SAT OC is highlighting prominent members of the wider aerospace community in the Diversity Corner.
AIAA Announcements AIAA Standards Under Revision
The following AIAA Standards are under revision: AIAA S-111 (Qualification and Quality Requirements for Space Solar Cells), AIAA S-112 (Qualification and Quality Requirements for Electrical Components on Space Solar Panels) and S-113 (Criteria for Explosive Systems and Devices on Space and Launch Vehicles). If you are interested in any of these revision projects, please contact Nick Tongson.
AIAA Committees Integrating Mission Objectives, Capabilities, and Technologies — 2022 SSTC Essay Contest Winners Announced
The AIAA Space Systems Technical Committee’s (SSTC) annual middle school essay contest continues to advance the committee’s commitment to directly inspire students and to involve local AIAA sections in educational pursuits. Each year, local sections sponsor parallel contests to feed into selection of national award winners recognized by the SSTC.
Seventh and eighth grade students were invited to participate. This year, AIAA local sections from across the country submitted entries to the contest. Participating sections included Cape Canaveral, Connecticut, Long Island, Los Angeles/Las Vegas, Palm Beach, Rocky Mountain, and Southwest Texas. For each grade, there were first-, second-, and third-place winners, which included $125, $75, and $50 awards for the students, respectively. The six students also received a one-year student membership with AIAA. The 2022 essay topic was “Describe a space mission that integrates at least three of the following system capabilities: autonomous systems; disaggregated satellites or platforms; on-orbit servicing, assembly, and manufacturing; in-situ resource utilization; small satellites; data analytics; optical and radio communications; advanced propulsion, advanced sensors (low mass, high-sensitivity, quantum, etc.). What is the objective of this mission, and how will the mission achieve the objective?”
The first-place winner for 8th grade is Lea Segal of Rancho Palos Verdes, CA (Los Angeles-Las Vegas Section). The second-place winner for 8th grade is Michael Mikati of Stuart, FL (Palm Beach Section). The third-place winner for 8th grade is Alayna Garrett of Colorado Springs, CO (Rocky Mountain Section).
The first-place winner for 7th grade is Axel Anderson of Colorado Springs, CO (Rocky Mountain Section). The second-place winner for 7th grade is Santiago Gollarza of Palm Beach Gardens, FL (Palm Beach Section). The third-place winner for 7th grade is Sid Patsamatla of Merritt Island, FL (Cape Canaveral Section).
The 2022 winning essays can be found below. The topic for 2023 is “Choose one aspect of the James Webb Space Telescope, describe how it works, and explain why it leads us to new discoveries and to answer important questions about the universe.” If you, your school, or your section would be interested in participating in the 2023 contest, please contact Anthony Shao-Berkery, Erica Rodgers, or your local section for more details.
AIAA Committees Describe a Space Mission Designed to Achieve a Specific Objectives (1st Place, 8th Grade)
Scientific Outpost in the Saturn System
Lea Segal, Palos Verdes Intermediate School
Teacher: Lyndsey Kier
One of the most fascinating places in our Solar System is planet Saturn, its rings and its eighty-two moons. The diversity of Saturn’s moons is mind boggling. There is massive Titan, the second largest moon in the solar system, and the only moon with an atmosphere. It has been observed to have an active climate with winds, rain and changing geology.[1] There is heavily bombarded Mimas, whose largest crater makes the moon look like the “Death Star.” There is Tethys, with its mysterious red arches, waiting to be explored. There is Enceladus whose vast ocean under the ice shell was found to have organic molecules and possibly harbor life. [2]
Exploration of the Saturn System has immense potential to significantly improve human knowledge, technology, and inspire future endeavors, as well as provide the answer to the question of the origin of life. I propose a mission to establish a scientific colony on Enceladus. The mission objective is to explore the moon’s geological features, further study organic compounds, and gain experience living and conducting research in space! During Phase One of the mission, a reliable source of energy will be established. Phase Two will consist of creating a robotic base on the moon. Finally, Phase Three will begin human exploration.
A large rocket, such as SLS or Falcon Heavy could be used to deliver Phase One equipment to the orbit of Enceladus. Similarly to the Cassini mission, a series of gravity assists around inner planets and Jupiter could be performed by the mothership spacecraft. Once the Saturn System is reached, the mothership will enter the orbit of Enceladus under its own propulsion. The orbiting mothership will be used as a communication relay between the surface and Earth. The mothership will deploy a series of highly specialized small satellites. These satellites, the scouts, will be equipped with cameras and radars to search the surface of Enceladus for a suitable landing site for establishing the future base. Based on the data previously collected by Cassini spacecraft, the south pole region of the Enceladus will be favored as it contains the thinnest (~6km) and warmest ice sheet and the most active geology.[3] The scouts will autonomously analyze and report data to the mothership, which in turn will deploy the first batch of landers to a specified location. Small autonomous landers will land on the surface. The job of the landers will be to establish power sources for the future base. One of the major problems about operations in the Saturn System is finding a reliable power source. Enceladus receives ~91 times (15W/m2) less power from the Sun than Earth and ~39 times less than Mars, which rules out the use of solar panels for this mission.[4] The Radioisotope Thermoelectric Generators will be used to provide power for the mothership, orbiting CubeSats and initially for the rovers. The rovers will lay large coils of electrical wires on the surface. As Enceladus orbits Saturn, it passes through Saturn’s magnetic field, effectively moving the coils of wires through the field. This will generate reliable and unlimited electrical power for the mission. In later Phases of the mission, hydro power could be used to supplement the electrical needs of the colony. Saturn’s gravitational pull applies tidal forces on the ocean. The water will move the turbines and generate electricity.
During Phase Two, another rocket will bring landers that will specialize in construction. They will take advantage of the power source established in Phase One to melt through the ice and build under ice shelters. The thick layer of ice around the shelter will protect sensitive equipment and ultimately humans from the space environment (vacuum and meteors) and solar radiation captured by Saturn’s magnetic field. Once the shelter construction is complete, the autonomous rovers could proceed to the manufacturing in-situ step of the mission. Using electric current, the ice of Enceladus can be first melted into water, then broken down into hydrogen and oxygen using electrolysis. This simple approach will allow creation of rocket fuel for exploring the Saturn System and oxygen for human needs in the future Phase. Enceladus offers a great base for moon hopping. It is a small moon with gravity 88 times less than Earth.[5] Specialized Phase Two robotic moon-hoppers will be refueled with the hydrogen and oxygen obtained by electrolysis as described above. They will be launched towards Titan, Mimas, and Tethys without a significant amount of fuel consumed (due to Enceladus’s low gravity). They will collect samples which can easily be returned to the base for further investigation and autonomous analysis. In addition, the rovers will lay wires in the habitat. This can provide electricity and warmth. The ice will be insulated to prevent melting and will also store heat. To assist the autonomous rover navigation around the surface of Enceladus, beacons can be installed at various locations around the base.
Finally, Phase Three will begin human exploration of the Saturn System. A not yet developed, more powerful rocket would have to be used (perhaps SpaceX’s Starship) to deliver human explorers to Enceladus in a timely manner. On Enceladus, humans will begin expanding the base by building additional under ice habitats, including areas for greenhouse farming. Next, crewed missions to other moons in the system will be conducted (taking advantage of plentiful rocket fuel mined in Phase Two and low gravity of Enceladus). Enceladus lacks important building material, such as rocks and metal. Astronauts will be tasked to collect these materials on the other moons, for future uses. Astronauts will begin drilling and melting though the ice sheets to explore Enceladus’s ocean in hopes of finding organic compounds and possibly life.
The proposed mission will improve human comprehension of the universe and potentially inspire billions of people on Earth.
References:
1. JPL. https://www.jpl.nasa.gov/news/the-first-global-geologic-map-of-titan-completed
2. JPL. https://www.jpl.nasa.gov/videos/ingredients-for-life-at-enceladus
3. US National Academy of Science. https://www.pnas.org/doi/10.1073/pnas.2011055117#
4. Photovoltaics Education ASU. https://www.pveducation.org/pvcdrom/properties-of- sunlight/solar-radiation-in-space
5. Wikipedia. https://en.wikipedia.org/wiki/Enceladus
AIAA Committees Describe a Space Mission Designed to Achieve a Specific Objectives (2nd Place, 8th Grade)
Space Debris: Solving the Problems of Today to Mitigate the Catastrophes of Tomorrow
Michael Mikati, Aerospace and Innovation Academy
Teacher: Shawna Christenson
Over one million pieces of space debris orbit Earth — each at thousands of miles per hour. Each piece, regardless of size, poses some variance of threat to operating space missions. Over the past few years, countless missions and millions of dollars have been victims to the ever- growing space debris, which only seems to get worse. This proposed satellite mission aims to eliminate space debris of various sizes utilizing aerogel deployables and a mesh nylon mesh. In order to achieve this goal, the mission will utilize optical and radio communications, advanced propulsion, and advanced sensors to properly locate and eliminate space debris.
Space debris is generally created during rocket launches and when non-functional satellites collide with each other, creating thousands of pieces of space debris in the aftermath. However, many non-functional satellites in orbit which have not already collided pose great danger due to their speed and size. The Natural History Museum explains that there are currently 128 million pieces of space debris larger than 1 millimeter, 34,000 pieces larger than 10 centimeters, and 3,000 out of 5,000 orbiting satellites are not functioning (O’Callaghan). Given these statistics the Museum determined that there is a 1 in 10,000 risk of collision that will require advanced maneuvers to avoid.
Current mitigation technologies of space debris are very preliminary and few missions on the issue have actually been tested. Proposed solutions include but aren’t limited to lasers, nets, harpoons, magnets, robotic arms, etc. Although these propositions have their benefits, some pose safety dangers while others are not compatible with different pieces of space debris. Another way of mitigating space debris is through lowering the satellite into earth’s atmosphere once it stops working, meaning the mechanisms will be built into the satellite. A group of researchers at Embry-Riddle Aeronautical University proposed to mitigate space debris is through implementing a solar sail on a satellite right before it stops working where the solar sail is directed in such a way the satellite will eventually slow down and burn up in the atmosphere (Pinholster). Although solutions like this may be able to reduce the amount of new space debris, there are no current solutions to eliminate existing space debris.
The proposed satellite aim to eliminate debris of various sizes utilizing aerogel deployables and a nylon mesh. The satellite will be powered and directed using a solar sail. The solar sail will enable the satellite to move in other directions outside of the original orbit based on the direction of the solar sail. The solar sail will be located on the front face of the satellite in relation to the axis of travel and will function as the primary source of energy and propulsion. In order to collect smaller pieces of space debris, aerogel deployables will be used. These deployables will function on the four side faces of the satellite. The aerogel deployables will deploy at right angles on the satellite perpendicular to the direction of travel. The aerogel deployables will have two metal rods on the right and left sides as well as smaller support metal rods in the aerogel to support them. Inside the satellite the aerogels will be stacked on each other, so they are slightly compressed and have enough force to be deployed. Once in the satellites desired orbit, the metal rods with aerogel will deploy out the four side of the satellite. These aerogels will be tested to collect and hold smaller pieces space debris. In order to test if space debris is collected a light in the left rod will be shown and on the right rod there will be a photoelectric sensor to record the light levels. If the light levels drop in a certain area of the aerogels, it can be presumed that a piece of debris is caught. This mechanism will be repeated for all four-aerogel deployable. The second part of the mission is to eliminate larger pieces of space debris using a nylon mesh. Nylon is very durable and has flexible properties which can accommodate for different sizes of space debris. This nylon mesh will be located on the rear face of the satellite based on the satellites axis of travel. On the end of the mesh there will be a circular opening for space debris to enter and the mesh to encapsulate it by closing down the opening. To find pieces of space debris, the satellite will use advanced sensors to larger object in orbit around earth. Then using a database and communication network it will determine if the satellite is functioning or if its mission is over. If so, the satellite will ask the ground station for an okay to remove the non-functioning satellite. Once the space debris is in the nylon mesh, using the solar sail, the satellite will adjust its orbit to get closer to earth’s atmosphere, then pointed at the atmosphere the closed part of the mesh will open and eject the space debris to burn up. In the case that the solar sail, which acts as propulsion, does not work back up thruster will be used to complete the mission. To eject the space debris, a square plate will lurch the object out of the mesh and into a slow burn in earths atmosphere. After the object is released into the atmosphere, the satellite will return to its desired orbit to find another large piece of debris.
Space debris has been a visible problem for the last few years, and with the further development of space exploration, this problem can only become more prevalent. This mission provides a solution to remove large and small pieces of debris through a power efficient and self- sustaining method via a solar sail. It is time we take the necessary action and testing today to preserve the ability of space travel and research of the tomorrow.
Works Cited
O’Callaghan, Jonathan. “What Is Space Junk and Why Is It a Problem?” Natural History Museum, Natural History Museum, https://www.nhm.ac.uk/discover/what-is-space-junk- and-why-is-it-a-problem.html.
Pinholster × Ginger Pinholster Assistant Vice President of News/Research Communications 386- 226-4811 pinholsv@erau.edu , Ginger. “Solar-Propelled Invention Designed to Clean up Space Trash.” Embry-Riddle Newsroom, Embry-Riddle Newsroom, 17 June 2021, https://news.erau.edu/headlines/solar-propelled-invention-designed-to-clean-up-space- trash#:~:text=A%20sail%20that%20unfurls%20itself,litter%20space%2C%20NASA%20h as%20reported.
AIAA Committees Describe a Space Mission Designed to Achieve a Specific Objectives (3rd Place, 8th Grade)
Journey to the TRAPPIST-1 System Propelled by Hydrogen Fusion
Alayna Garrett, Eagleview Middle School
Teacher: Elizabeth Busler
The search for extraterrestrial life has always been a driving force for the human race. The idea that we aren’t alone in this universe is the ultimate motivation for the research and innovations we come up with. Recently, telescopes have spotted a system with seven exoplanets orbiting an ultra-cool red dwarf star. This system, called the TRAPPIST-1 system—named after the telescope that spotted it—has several conditions that makes it an excellent destination for space travel. To reach the system successfully, autonomous systems, advanced propulsion, and in-situ resources will be utilized.
The TRAPPIST-1 system is just under 40 light years away. Out of the seven exoplanets orbiting the ultra-cool red dwarf star, three are in the Goldilocks zone for this particular system. The planets are thought to be similar in structure to Earth, with similar sizes, gravities, and densities. The farthest planet from the star, TRAPPIST-1H, is closer to the star than Mercury is to the Sun. The planets are remarkably close together compared to our solar system, and have extremely short years, with the longest being 19 days. They are all thought to be tidally locked, due to their proximity to the star. Although the planets aren’t quite Earth’s twins, they are exceptionally similar in many aspects. TRAPPIST-1E, F, and G orbit in the system’s Goldilocks zone. This is the distance from the star where liquid surface water is most likely to be found. This system is one of the most promising places where many of the conditions for supporting life are met.
Some scientists theorize that the planets might hold alien life. It would be an incredible discovery if there was indeed life in this system, no matter how primitive. The main objective of this mission is to gather more information on the planets, specifically TRAPPIST-1E. This planet has been studied most and is most likely to have easily accessible liquid water. The probe will bring a rover to touch down on the surface of the planet. Soil samples will be collected to later be brought back to Earth to be analyzed. The rover will look for water, liquid or ice, on the surfaces, and will carry technology to identify the composition of the planet’s atmosphere to see if it contains the gasses required for life known on Earth.
The distance between Earth and the TRAPPIST-1 system will render normal communication methods unusable. From Earth, we will not be able to give the rover real time instructions, so it will need to be able to completely self-direct itself. The rover is going to be equipped with an advanced version of NASA’s AutoNav system. “…this enhanced system makes 3D maps of the terrain ahead, identifies hazards, and plans a route around any obstacles without additional direction from controllers back on Earth” (Brennan, 2021). This system will allow the rover to make its own decisions on where to explore, limiting the amount of human interaction required. As this planet has not been explored previously, the rover will be in completely new territory. No photos exist of the surface, so it will be mapping its own course and analyzing the best site to extract samples.
Because the TRAPPIST-1 system is 40 light years away, it is going to take a while to get there. It would take an extraordinary amount of thrust to get up to even 10% of the speed of light, so more advanced thrust systems are going to be a must. With current technology, it will be impossible to carry the amount of fuel needed to travel all the way to the system. Instead, a propulsion system using hydrogen that can be refueled using any star or gaseous planet matter will be used on this mission. Using a nuclear fusion reactor similar in design to a stellarator, deuterium particles will be fused under extreme heat. In a doughnut-shaped reactor, magnetic field coils will contain ionized plasma heated to nearly 100,000,000 degrees Celsius (Clery, 2015). The plasma will heat the deuterium enough to cause deuterium-deuterium fusion. This reaction releases intense amounts of energy that can be used to power the spacecraft. There will be a reactor at both ends of the probe, to aid in slowing the craft down when it gets closer to its destination.
To make the trip back from the TRAPPIST-1 system, the probe will need to refuel and restock on its hydrogen reserves. This mission will last for 2190 days, or 6 years, after arrival. While the rover is exploring the surface of the planet, the probe will collect hydrogen from the solar wind particles the star sends out. After the 2190 days are up, the probe is programmed to retrieve the rover, which will use a miniature thrust system of its own, using regular rocket fuel, to get off of the planet’s surface. The rover will then be magnetically attracted to the probe and docked into it, similar to how space shuttles dock into the ISS.
Although there are destinations in space that are far closer than the TRAPPIST-1 system that we could explore, the system remains unique in that every planet in it has striking similarities to Earth. This system may soon provide a home to life forms from Earth if this mission proves to be successful. With the advances in technology that we have made, future interstellar and intergalactic missions to explore the universe and look for alien life will be possible.
Works Cited
Bigot, Bernard. “Hydrogen Fusion: The Way to a New Energy Future.” The European Files, 11 Oct. 2021, https://www.europeanfiles.eu/environment/hydrogen-fusion-the-way-to-a-new-energy-future.
Brennan, Pat. “NASA’s Self-Driving Perseverance Mars Rover ‘Takes the Wheel’.” Edited by Tony Greicius, NASA, NASA, 1 July 2021, https://www.nasa.gov/feature/jpl/nasa-s-self-driving-perseverance-mars-rover-takes-the- wheel.
Clery, Daniel. “The Bizarre Reactor That Might Save Nuclear Fusion.” Science, 21 Oct. 2015, https://www.science.org/content/article/bizarre-reactor-might-save-nuclear-fusion#:~:text=Stellarators%20face%20the%20same%20challenge,temperature%20of%20the%20sun
%27s%20core.
Harris, William. “What If You Traveled Faster than the Speed of Light?” HowStuffWorks Science, HowStuffWorks, 8 Mar. 2018, science.howstuffworks.com/science-vs-myth/what-if/what-if-faster-than-speed-of-ligh t.htm.
Kaser, Rachel. “NASA Discovered Seven Nearby Planets That Could Support Life.” The Next Web, The Next Web, 23 Feb. 2017, thenextweb.com/space/2017/02/22/nasa-discovered-seven-nearby-planets-that-could- support-life/.
Lanctot, Matthew. “DOE Explains…Deuterium-Tritium Fusion Reactor Fuel.” Energy.gov, https://www.energy.gov/science/doe-explainsdeuterium-tritium-fusion-reactor-fuel.
“Nuclear Fusion Power.” Nuclear Fusion : WNA – World Nuclear Association, Aug. 2021, https://world-nuclear.org/information-library/current-and-future-generation/nuclear-fusion-power.aspx#:~:text=With%20current%20technology%2C%20the%20reaction,4%20Me V%20for%20D%2DD%20fusion).
Peshin, Akash. “How an Innovative Propulsion Technology Will Send You to Mars in 3 Days?” Science ABC, 4 Jan. 2022, https://www.scienceabc.com/innovation/what-is-photonic-propulsion-lightsail-2-solar-sai l.html.
AIAA Committees Describe a Space Mission Designed to Achieve a Specific Objectives (1st place, 7th Grade)
Mars Sample Return Mission
Axel Anderson, Eagleview Middle School
Teacher: Mrs. Jacquet
Right now, there is a rover on Mars called Perseverance that is collecting rocks and other samples from Mars, searching for signs of life and other secrets of the solar system. These valuable artifacts will remain on Mars unless we retrieve them. Fortunately, a mission has been proposed, called the Mars Sample Return Mission to do just that [1]. Although plans have been discussed, they are only in their early stages. To accomplish this mission, NASA will have to send another spacecraft to Mars, which will enter the Martian orbit, deploy a smaller lander to the surface, load the samples from Perseverance and onto an ascent vehicle, which must shoot up to the spacecraft, and finally return to Earth for study. This process is complicated and will need the aid of many advanced technologies. Three of these are advanced propulsion, autonomous systems, and advanced sensors. Scientists and Engineers are working hard to improve these technologies for this mission. These advancements will also aid the exploration of the entire solar system and hopefully beyond.
One of the technologies needed to return samples from Mars is advanced propulsion. This will reduce travel time and use less rocket fuel. For a spacecraft to go all the way to Mars and back, it will take so much fuel that the rocket might not even be able to lift off the ground. An alternative is to gather energy for propulsion while it travels through space. Thankfully, this is possible with electric propulsion. Electric propulsion allows the ship to accelerate on the journey there, which is much faster than coasting all the way to Mars and back, as we do with rocket propulsion. Fuel can be collected from the sun’s energy as the ship travels.
The NASA article, “The Propulsion We’re Supplying, It’s Electrifying”, explains that we can take energy from the Sun and use it to power thrusters [2]. The energy is used to ionize gas propellants and fire them out of the thrusters using electric or magnetic fields at ludicrous speed (not quite as fast, closer to light speed) while emitting a pleasant light blue glow. NASA has already demonstrated the use of Electric propulsion with the Dawn mission [3]. Once the Dawn spacecraft entered space, it fired three ion thrusters with Xenon gas fuel and accelerated to Vesta. As it arrived, it slowed itself down by turning around and firing the thrusters to enter the orbit. After orbiting for a year, it fired its thrusters once more and traveled to Ceres to orbit. These maneuvers were only possible by the use of electric propulsion. While these thrusters only generated a small force (0.33 oz.) they could accelerate Dawn all the way up to 60 mph in four days [3]. That may sound slow, but over the course of its eleven-year mission, it can get a lot of speed in the vacuum of space.
Another helpful technology NASA should use is autonomous systems. Autonomous means having the ability to control oneself, rather than being controlled. A system or robot can do this by collecting information from onboard sensors and following prewritten programs to make decisions and act on its own. Buttons do not have to be pressed for it to move. This is very similar to my First Lego League robot which cannot be teleoperated. Autonomy is essential for the different machines participating in this mission because of how far they will be from Earth. Radio signals from Earth to vehicles on Mars can take 5 to 20 minutes to arrive. This is way too long to effectively teleoperate a robot. By using autonomy, the robot would not have to wait several minutes for guidance from humans.
One part of the mission that would have to be autonomous is the descent to the Martian surface. This is the most dangerous part of the mission with several very complicated steps. These steps must be completed perfectly, at the right time or the spacecraft will plummet into the surface of Mars. It would be nearly impossible to control every step remotely because of the time it takes to communicate. For example, when perseverance landed on Mars, it used “Terrain Relative Navigation,” [4] a type of computer vision. This uses a camera to see different landmarks on the surface of Mars to find its location and recognize a pre-determined landing site. Scientists and engineers have been improving computer vision with machine learning, through the use of neural networks [5]. Neural nets are used to train computers to recognize things or complete a task, by showing the computer several images that the computer can learn from. NASA should consider using neural nets to make the landing more reliable. A neural net would be much more responsive to unexpected conditions, like bad weather, and could find its own landing site, without the aid of pre-selected sites, which could have changed.
The final technology is advanced sensors. Sensors are to all space missions, but are critical to the use of autonomous systems. The Lander Vision System uses a camera, Inertial Measurement Unit, and Flash Lidar, all of which are advanced sensors constantly being improved [6]. There are also many other helpful sensors that can gather data to improve location or orientation information. Another useful sensor is a Sun sensor [7] which is used in groups of sixteen to determine sun visibility. This is needed to ensure the solar panels face the sun to collect energy for electric propulsion, otherwise the ion thrusters will not have the power needed to run. Overall, improved sensors will increase the odds of a successful sample return mission.
The possibility of returning specimens from Mars is very exciting and inspiring. As we study the specimens on Earth, we will learn more about the solar system and how it was created. This mission is difficult, but it is still possible. Through the use of the three technologies advanced propulsion, autonomous systems, and advanced sensors, this mission can finally be accomplished with ingenuity and hard work!
References
1. Jet Propulsion Laboratory. “Mars Sample Return – Mars Missions – NASA Jet Propulsion Laboratory.” NASA, NASA, https://www.jpl.nasa.gov/missions/Mars-sample-return-msr.
2. Sands, Kelly. “The Propulsion We’re Supplying, It’s Electrifying.” NASA, NASA, 16 Oct. 2020, https://www.nasa.gov/feature/glenn/2020/the-propulsion-we-re-supplying-it-s- electrifying.
3. “Dawn Spacecraft.” NASA, NASA, 12 Dec. 2018, https://solarsystem.nasa.gov/missions/dawn/technology/spacecraft/.
4. O’Neill, Mike. “Entry, Descent, and Landing: The Most Intense Phase of the Mars Perseverance Rover Mission.” SciTechDaily, 11 Feb. 2021, https://scitechdaily.com/entry-descent-and-landing-the-most-intense-phase-of-the-Mars- perseverance-rover-mission/.
5. Upadhyay, Yash. “Computer Vision: A Study on Different CNN Architectures and Their Applications.” Medium, AlumnAI Academy, 29 Mar. 2019, https://medium.com/alumnaiacademy/introduction-to-computer-vision-4fc2a2ba9dc.
6. Johnson, A., and Golombek, M., “Lander Vision System for Safe and Precise Entry Descent and Landing,” Jet Propulsion Laboratory, California Institute of Technology.
7. “Sensors.” NASA, NASA, https://Mars.nasa.gov/mro/mission/spacecraft/parts/gnc/sensors/, 14 Mar. 2022.
AIAA Committees Describe a Space Mission Designed to Achieve a Specific Objectives (2nd place, 7th Grade)
Space Mission to Enceladus
Santiago Nicolas Gollarza, Aerospace and Innovation Academy
Teacher: Shawna Christenson
In the future, a manned space mission to Enceladus, a moon of Saturn, could be possible for research. To explore Enceladus safely & quickly, however, several technologies must be used, such as small satellites, advanced propulsion, and optical & radio communications to have the mission succeed. This mission to explore Enceladus will benefit humans as we search for other planets and possibly to find life.
Research missions are vital to NASA, and research on the planet Saturn and its moons are no exception. One of the more notable of these moons is Enceladus, which is an icy moon of Saturn with large amounts of geysers on the surface, spewing out ice and water vapor into Saturn’s orbit. Recently, however, the Cassini probe has found liquid saltwater under the surface. The conditions in this ocean replicate conditions on the early earth, as water is one of the hallmarks of organic life on the earth, allowing many astronomers and biologists to theorize that this moon may contain organic life. The European Space Agency has discovered that “Enceladus expels around 250 kg of water vapor every second, through a collection of jets from the south polar region known as the Tiger Stripes because of their distinctive surface markings” (2011). The discovery of water under Enceladus’ frozen ice surface means that research missions could be very productive in finding evidence of organic chemicals similar to those under Antarctica’s ice sheets.
The mission will have to be long term as Enceladus is 790 million miles from Earth, in Saturn’s orbit. This would require very powerful, yet energy efficient propulsion systems. One type of system which is being experimented with is ionized xenon gas, which uses the element xenon. Because xenon gas is inert and relatively light, it would be easy to store in a ship on the long term with little risk of failure. Ion thrusters have been used before with productive results.
According to NASA’s Michael Patterson “Ion thrusters (based on a NASA design) are now being used to keep over 100 geosynchronous Earth orbit communication satellites in their desired locations”(2016). Since Saturn is near Jupiter, a gravitational assist could help the mission to conserve fuel. Gravitational assists have been used before, such as with the Cassini probe. The trip would take around 8 years. Advanced propulsion systems can allow for heavier payloads, or faster times to get to the moon, and can be used on a mission with great efficiency.
Another technology that can help is small satellites. Small satellites are any satellite less than 180 kilograms and are easier to produce than large satellites due to their small nature. Smallsats could be used to monitor atmospheric conditions, a necessity for any manned spacecraft planning to land, as it can monitor the weather. According to Elizabeth Mabrouk at NASA, “CubeSats now provide a cost-effective platform for science investigations, new technology demonstrations and advanced mission concepts using constellations, swarms and disaggregated systems” (2015).
Small satellites can incorporate sensors such as infrared & temperature sensors. This will help reduce the cost of a space mission, and can help pre-diagnose issues before they occur, along with monitoring conditions during a mission. CubeSats are very common in use in the current day; they are used for atmospheric monitoring & experiments on the earth, and many institutions use them for research.
A final technology which can be used to help space colonization is radio & optical communications. Optical communication uses light to transmit communications, while radio communication uses low-frequency waves. Optical communications can be made easier using small satellites. While radio communications travel through ice in some frequencies, it doesn’t pass through at higher frequencies. According to George Hale, at NASA’s IceBridge project, “Ice, on the other hand, reacts differently depending on the radar’s frequency. It reflects high- frequency radio waves, but despite being solid, lower frequency radar can pass through ice to some degree. This is why McCord’s uses a relatively low frequency—between 120 and 240 MHz” (2013). Radio waves can be used to communicate from earth to the moon, & from one place on the moon to another. The use of radio systems will prove beneficial in space missions to moons such as Enceladus.
The use of technologies such as advanced propulsion systems, radio and optical communication systems, & small satellites will benefit a future space mission to Saturn’s moon Enceladus. If humans are to ever explore the moons of other planets in the solar system, these technologies are necessary for any possible manned mission’s plans. These technologies are very reliable and have been used many times, so they should be used on a mission to Enceladus. A mission to Saturn’s moons will be helpful for finding clues on how life began on earth, and possibly on other planets.
Works Cited
European Space Agency. (2011, July 26) Enceladus Rains Water On Saturn. https://www.esa.int/Science_Exploration/Space_Science/Herschel/Enceladus_rains_water_onto_ Saturn
Hale, George. (2013, December 2) Airborne Radar Looking Through Thick Ice During NASA Polar Campaigns. https://www.nasa.gov/content/goddard/airborne-radar-looking-through-thick-ice-during-nasa- polar-campaigns/#:~:text=Radio%20waves%20travel%20through%20air,through%20ice%20to%20so me%20degree.
Mabrouk, Elizabeth. (2017, August 7) What are SmallSats and CubeSats? https://www.nasa.gov/content/what-are-smallsats-and-cubesats/
Patterson, Michael. (2017, August 7) NASA- Ion Propulsion. https://www.nasa.gov/centers/glenn/about/fs21grc.html
AIAA Committees Describe a Space Mission Designed to Achieve a Specific Objectives (3rd place, 7th Grade)
Time Travel and the Use of Convoluted Space Geometries
Sid Patsamatla, Edgewood Jr/Sr High School
Teacher: Katie Reninger
Time travel has long been an awaited goal, as mankind has been trying to greet it with outstretched arms for the past few decades. Time travel is the conceptual idea of moving through different points in space analogous to movement through certain points of time. It is hypothetical that machines called time travel machines can make this phenomenon turn into realism.
However, there can be another approach. We can create algorithms, complex sensors, and circuits for automated space vehicles, to emit energy that can turn into spacetime geometries such as wormholes and cosmic strings. These produce objects traveling from point a to point b, faster than the speed of light. Although, in today’s world, we do not have all the information to expedite a project like this fast enough for it to be in service for the upcoming few decades; the smart minds of scientists, physicists, and engineers can originate it, for actuality.
Physics has an immense amount of knowledge for the technologies theorized with convoluted spacetime geometries. Physicist Albert Einstein developed a theory of special relativity, which is deceptively simple. The theory connects space and time, at a constant speed and direction. In simple terms, it states that the speed of light is constant, there is nothing greater than the speed of light, and there is no “absolute frame of reference.” The frame of reference between two different objects is relative. An observer moving through space at a greater speed than another observer will experience time at a slower rate. A real-life example proves evidence for this. Astronaut Scott Kelly was born exactly 6 minutes before his brother Mark Kelly. Scott stayed in orbit for a total of 520 days while Mark spent only 54 days in space. This difference in speed in which they experience time increased their age gap by 5 milliseconds. Consequently, time dilation happens between humans, and GPS satellites, as well. According to special relativity, the faster an object moves relative to another object, the slower that first object experiences time. According to the American Physical Society, for GPS satellites with atomic clocks, this effect cuts 7 microseconds, or 7 millionths of a second, off each day. Even though Albert Einstein said that nothing is greater than the speed of light. That is probably mistaken, as two things are frankly proven to be faster. One of these things is spacetime geometries, called wormholes. Wormholes are shaped like two funneled black holes, on top of each other, that use gravity to make a shortcut through spacetime. Julian Sonner of MIT, finds that particles seem to be connected to a wormhole in 4-D space, while in 3-D space, that wormhole is only a string.
Ongoing, into the technological and engineering aspects of this project. First, we would need to construct an object that can see things in 4-d dimensional space, because the human brain cannot. We could manufacture a camera and multiple sensor systems, that uses super high-resolution computer vision, to detect hypercubes and observe their transformations, between different dimensions, such as n-1. We could also use quantum ghost imaging of many particles on these technologies to improve their photo resolution quality. Consequently, we can also use machine learning and artificial neural networks to feed in inputs of many simulations and pictures of objects in multiple dimension states, pool the image, and use complex software algorithms to get an output of where the wormhole is in x,y, z, w space. This is still not in our reach as a species to do this, but hypothetically may be an option shortly. Next, a quantum entanglement system needs to be manufactured. An idea to create this is to have a series of lasers horizontals from each other, and an array of crystals that extend from the front of the space vehicle, on a series of trays, and this will create an entanglement of particles, than can be separated by large distances, up to hundreds of miles. This is called the spontaneous parametric down-conversion (SPDC). This means that a highly concentrated region that will “suck in” things faster than the speed of light will emerge, which is also known as a black hole. If we repeat this multiple times, and two black holes are in a position in which they form a funnel then an electromagnetic wormhole could be created. Connected to the camera, would be a series of huge binary and quantum computers. Binary computers will be used for sending analytics, and data wirelessly down to satellite stations in Earth’s atmosphere and radio stations on Earth. The quantum computers could be programmed in Qiskit, in which they can store more data, and perform more complicated computations, about the data received by the sensor systems and camera.
In conclusion, a sustainable spacecraft or space vehicle would be established to create wormholes, using quantum physics, and advanced computers, software, and data transmissions. The main idea to create a wormhole is with the principle of quantum entanglement to create key photon pairs to create a black hole. Then if multiple photons are entangled, with a very low probability but still possible, two black holes will create a funnel between two different analogous points, in space. We then can use a camera, that uses neural networks and computer vision using multiple programming libraries, to spot this black hole, along different dimensions in 4-d which the human eye and brain can’t do. This camera will be connected along multiple lines of computers, that work on multiple states of logic and electronics such as binary and quantum to send data, and analytics using electromagnetic radiation, and satellites. This prototype may have many multiple challenges to overcome, but with the progression of the human species to overcome challenges and find solutions, there is still a little bit of light to do the impossible.
Obituary AIAA Fellow Gasich Died in January 2022
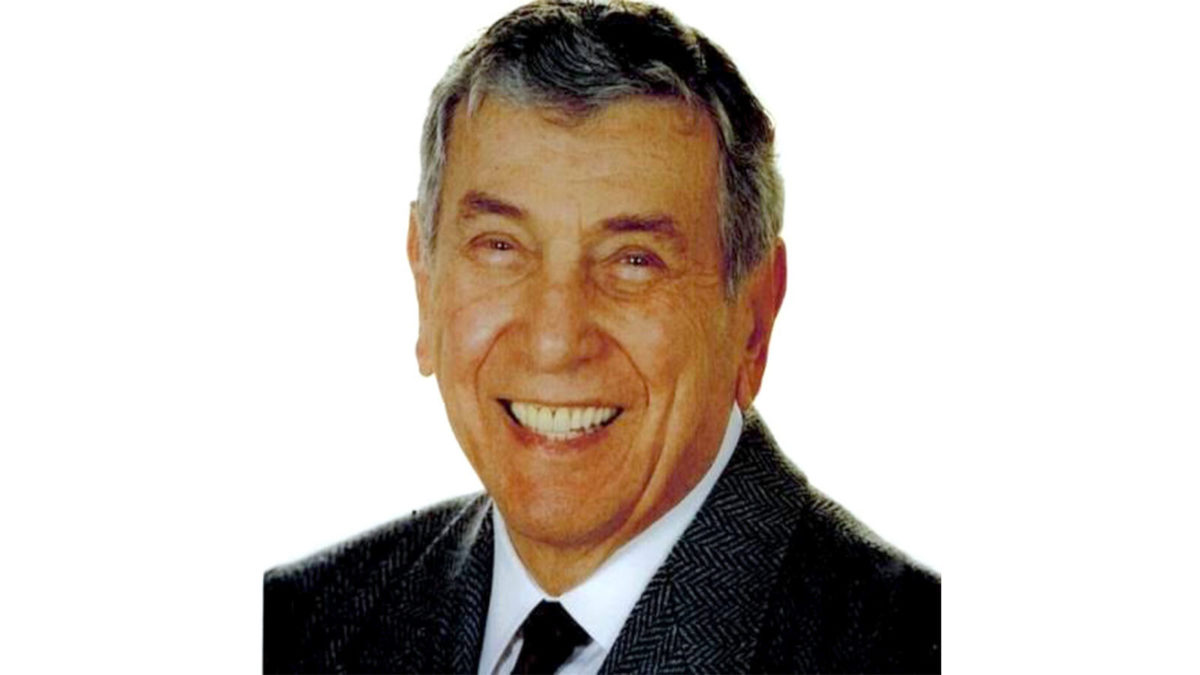
Welko E. Gasich died on 14 January 2022. He was 99 years old.
Gasich’s interest in aircraft was sparked at age 10 when his uncle gave him a flight in his airplane. He received his B.A. degree in Mechanical Engineering from Stanford University in 1943. His graduate studies were interrupted by World War II when he served in the United States Navy as a flight test engineer achieving the rank of Lieutenant. As an officer on detached duty with NACA, he was placed at Moffett Field to correlate the P-39 flight test results of drag divergence Mach number with wind tunnel tests. He also was the project engineer in charge of propeller tests on the XSB2D-1 single-engine airplane that eventually crashed due to engine failure. Both he and the pilot survived, but the airplane was destroyed. After his active duty in the U.S. Navy (1944–1946), he continued in the U.S. Naval Reserve until April1954, at which time he was honorably discharged.
Returning to Stanford University, he received his M.S. in Aeronautical Engineering in Aeronautical Engineering in 1948. Gasich also received a Professional Degree of Engineering from California Institute of Technology, and he was a graduate of the Sloan Executive Program, Graduate School of Business, Stanford University (1967).
Gasich began his professional career with Douglas Aircraft Company, El Segundo Division, where he worked in the fields of aerodynamics and aeroelasticity on a variety of aircraft. He also worked at the Rand Corporation, as Chief of Aircraft Design on various aircraft programs in support of the U.S. Air Force. Gasich was asked to join the Northrop Corporation as Chief of Preliminary Design in 1953. He had a major role in Northrop’s conception and development of the U.S. Air Force’s lightweight supersonic jet trainer, the T-38 Talon, of which he was a co-patentee. The T-38 entered service with the USAF. He was also intimately involved in the design of the F-5 (co-patentee) as well as the F-5A, and the F-5E supersonic fighters. In 1956 he was promoted to Director of Advanced Systems and in 1961 to Vice President of Engineering and Assistant General – Manager Technical.
Following his return to Northrop from the Sloan Executive Program at Stanford University, he was General Manager of Ventura Division. While there he managed subsystems such as the parachute recovery system for Mercury, Gemini, and Apollo space vehicles. In 1971 he returned to the Aircraft Division of Northrop as General Manager. He worked on the NASA M-2 and HL-10 space recovery vehicles as well as the A-9, YF-17, F-18 fighters and the early work of the B-2 bomber. Thereafter, he was appointed a Corporate Officer and Senior Vice President of Advanced Projects. In 1985 he was elected Executive Vice President of Programs until his retirement in 1988.
Gasich was elected to the National Academy of Engineering for his work on supersonic aircraft design and leadership in the engineering field. He was a member of the Joint Strategic Target Planning Staff (JSTPS) Scientific Advisory Group. He was also elected a Fellow of AIAA and SAE. He served as a member of the SAE Board of Directors and on various university engineering advisory boards. He was the author of various articles on technical subjects and engineering management. Upon his retirement from aerospace, he studied the key factor in determining the horsepower output of racing engines. After two years of research on Ferrari engines, he determined that bore/stroke ratio was a powerful index of engine performance, and he authored the book, Forty Years of Ferrari V-12 Engines, published by the Society of Automotive Engineers.
Obituary AIAA Fellow Boehm Died in August
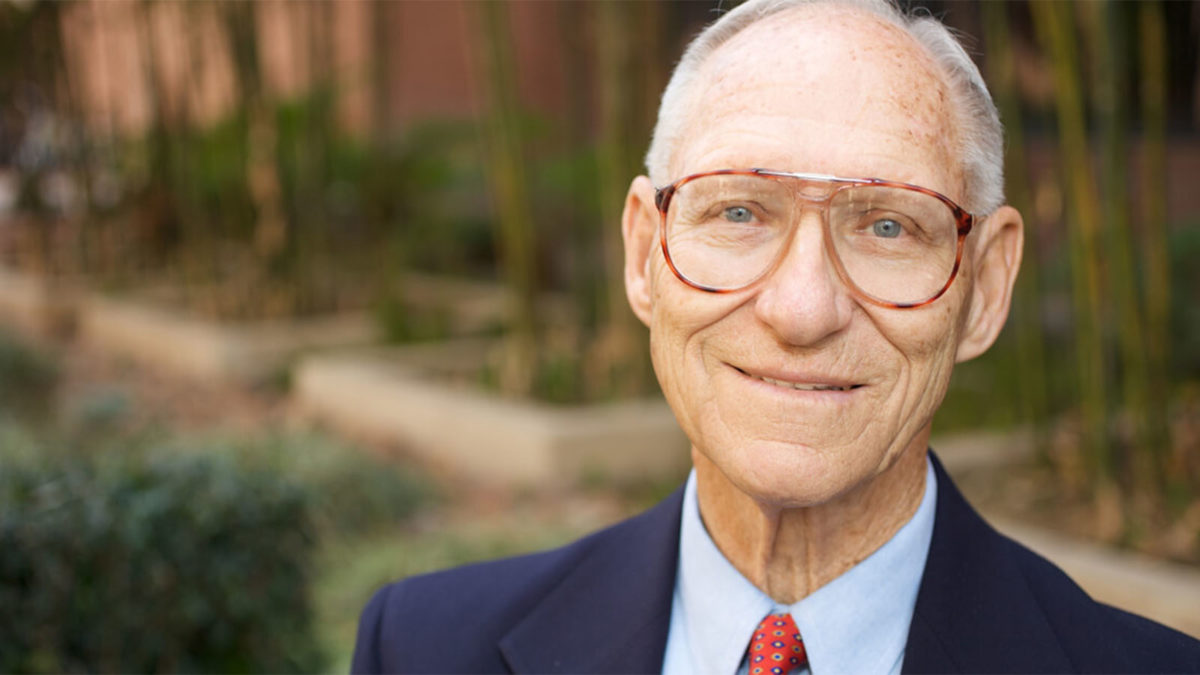
Barry Boehm, a pioneer in the fields of computer science and software engineering, died 20 August. He was 87.
Boehm earned his bachelor’s and master’s degrees from Harvard University and his Ph.D. in mathematics from the University of California, Los Angeles. He worked at the RAND Corporation, TRW Inc., and DARPA over the course of his career before joining the University of Southern California’s faculty in 1992. He served as a USC distinguished professor of computer science, industrial and systems engineering, and astronautics until he retired in May 2022.
He is best known for the invention of a software cost estimation model that transformed the discipline. This model allowed software engineers and project managers to gain control of projects that were increasingly behind schedule, over budget, and low quality, saving companies and government agencies untold billions of dollars. He described this Constructive Cost Model in the 1981 book, Software Engineering Economics.
Boehm also developed the spiral software lifecycle model, which recognized that large, expensive, and complicated projects require an iterative development process with gradual releases and refinement of a product through each phase.
Boehm was the author of more than 900 publications, including nearly 200 journal articles, 6 textbooks, and hundreds of conference papers, as well as presentations, keynotes, and webinars. He also helped guide more than 30 Ph.D. students at USC. In 2007, the International Conference on Software Engineering held a symposium to honor Boehm (“Software Engineering: The Legacy of Barry W. Boehm”), which highlighted his legacy by gathering colleagues and members of the software engineering community. The symposium also published a book that contained reprints of his 42 most influential papers. In 2014, his work was further discussed in the Journal of Cost Analysis and Parametrics article, “On the Shoulders of Giants: A Tribute to Professor Barry Boehm.”
Boehm received many awards and recognitions, including the 2018 SERC Founders Award and the 2019 INCOSE Pioneer Award “for his work as a systems pioneer uniquely contributing to the advancement of systems engineering through extensive research, education and the application thereof in industry.” He also received the 1979 AIAA Information Systems Award and the 2013 AIAA Aerospace Software Engineering Award. He was a fellow of the International Council on Systems Engineering (INCOSE), AIAA, the Association of Computing Machinery (ACM), and IEEE, and was elected as a member of the National Academy of Engineering in 1996.
Obituary AIAA Associate Fellow Tauber Died in October
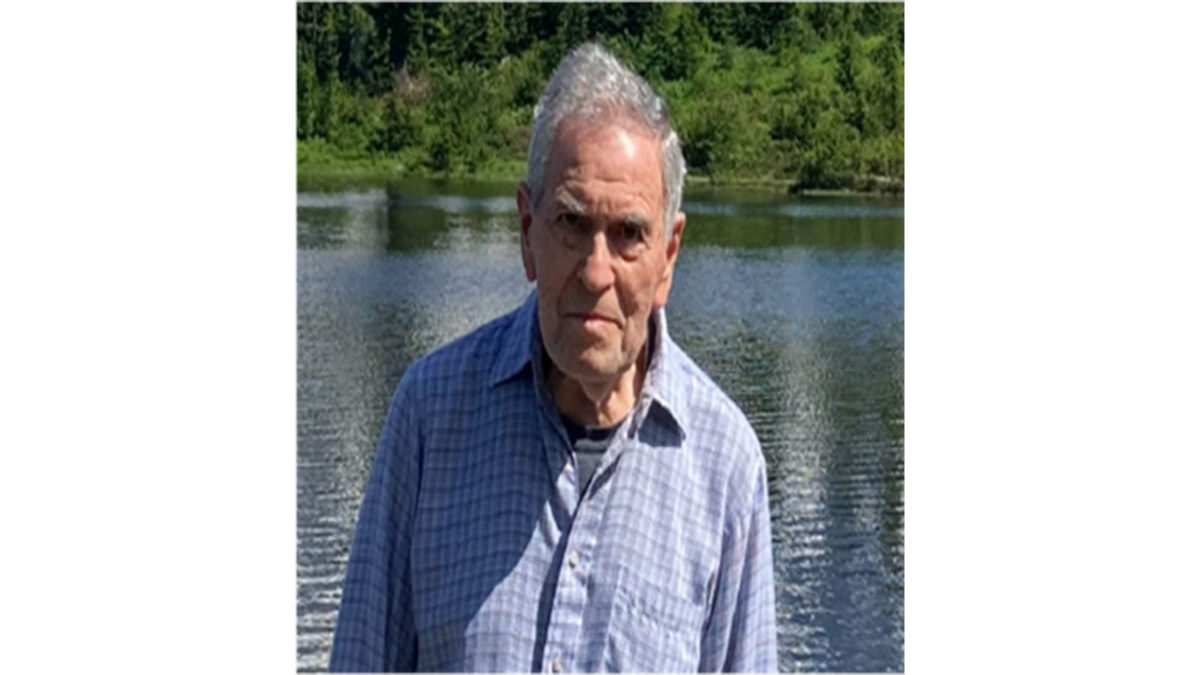
Michael E. Tauber died on 17 October 2022.
A survivor the Holocaust, Tauber was educated in Aeronautical Engineering at the University of Washington and Stanford University (1959). The majority of Tauber’s career was devoted to NASA’s programs as a civil servant starting at NASA Ames Research Center in 1962, until he retired from Analytical Mechanics Associated, Inc. in 2016.
One of Tauber’s most important works in space exploration was on the Jupiter Probe, which began in 1966. His study demonstrated that a human-made object could survive entry into the giant planet’s atmosphere and return data to Earth revealing information regarding its structure and composition down to about 100 bars. Features of the probe and its trajectory included the use of a carbon phenolic heat shield and a shallow entry angle in the direction of the planets’ equatorial rotation. The final results of Tauber’s feasibility study were presented at an AIAA meeting in fall 1970 and published in the Journal of Spacecraft and Rockets in 1971. On 7 December 1995, Tauber was able to see his findings successfully applied when Galileo’s entry took place on at a speed of 47.41 km/s; its entry is considered the most severe in the history of the space program. Recession sensors on board the probe showed that the thickness of the forebody heat shield was overestimated in the nose region and underestimated on the flank. Design tools used for the Galileo probe heat shield are primitive compared to those used by today’s entry technologists, but because of the complicated flow physics and ablation processes involved the entry remains a focus of study to completely understand the observed heat shield recession. Tauber was involved with the design and understanding of the Galileo probe’s entry performance, even in the latter stages of his career.
Tauber’s research relating to atmospheric entry included analysis of important Mars missions including Pathfinder, Mars Exploration Rover (MER), Phoenix, and Insight. He was also heavily involved in the development of the design of the Stardust Comet Sample Return heat shield. He developed tools such as the Tauber-Sutton correlation for entry heating and explanations of why probe designs depend upon the composition of the planetary atmosphere under consideration. Tauber was the 2010 winner of the coveted International Planetary Probe Workshop’s Alvin Seiff Award “In recognition of his contributions over the past fifty years as an Entry System Engineer, as a teacher and in developing concepts and successfully leading thermal protection systems (TPS) and entry system design of such missions as Galileo and Mars Pathfinder.” Tauber is one of the greatest contributors to NASA’s planetary missions, aerothermodynamics, and applications of thermal protection systems.
He was asked to contribute to NASA’s program in rotorcraft from 1971 to 1985. He also excelled in this area, winning the 1985 Howard Hughes Award, “For his contributions to the development and application of ROT22, the first three-dimensional full potential computer code capable of predicting the transonic flow around helicopter rotor blades.”
In addition to his contributions as a NASA researcher, Tauber also devoted considerable time as a teacher and a mentor to two generations of aerospace engineers. He taught courses in engineering at Stanford University, Santa Clara University, North Carolina University, and the University of Tennessee.
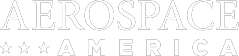