Test time for optical comms
By Debra Werner|February/March 2021
Fiber might not have reached your neighborhood, but anyone who does business in space will need a wireless version in the cosmic neighborhood, and soon. After decades of experimentation, 2021 could become the long-sought pivotal year for optical communications in space, notwithstanding an early setback. Debra Werner tells the story.
★ Click at right to open this story’s Knowledge Guide entries.
★ Click star at right to open Knowledge Guide.
In the space business, bad news sometimes arrives in dry language. Two mini-fridge-sized experimental optical communications satellites that were supposed to reach orbit in January “were damaged during launch processing at Cape Canaveral,” DARPA confirmed weeks before launch in a brief statement.
If or when the satellites, Able and Baker, reach low-Earth orbit, they’ll bounce invisible near infrared laser light encoded with telemetry between each other via intersatellite links, a challenge that requires precise attitude control and laser pointing. This ambitious optical demonstration is part of DARPA’s Blackjack program to demonstrate a high-speed communications constellation in low-Earth orbit. It also could have broad civilian applications, and its lessons could apply to the Pentagon’s proposed “Transport Layer” of communications satellites. Military commands, intelligence collections and more would bounce through space over this layer and to users on the ground at rates up to 100 times faster than with today’s radio frequency satellites.
“Optical is really good at building a point-to-point, high-bandwidth connection,” says Stephen Forbes, DARPA Blackjack program manager. “That’s where I see the immediate sweet spot for optical, connecting nodes that need to share a lot of data, whether that is from satellite to ground or from ground to ground through a satellite.”
If these broadband rates were once viewed as a mere convenience, they could soon become a must-have, and for more than military and intelligence applications. Miniaturized electronics and falling launch prices for small spacecraft are boosting the numbers of satellites in low-Earth orbit and making factories and mining operations suddenly seem feasible in some opinions. In deep space, NASA is planning its series of Artemis human missions to the moon, and Elon Musk wants to colonize Mars.
With the future of Able and Baker now in question, hopes among optical technologists for a pivotal year in optical communications that could impact all those users have shifted to another upcoming launch, this one under NASA’s Laser Communications Relay Demonstration, or LCRD, project. A payload consisting of two optical telescopes and communications electronics will receive and transmit data-encoded laser light while operating from the latest of the U.S. Space Force-managed (formerly Air Force-managed) Space Test Program satellites, due for launch in late February. If all goes as planned, the telescopes will bounce video, images, voice and text among ground stations, low-Earth orbit satellites and also the International Space Station at rates as high as 1.2 gigabits per second, or about 10 times as fast as the Wi-Fi setup in the typical U.S. home. The experiment will be a step in NASA’s plan to expand optical communications to deep space, especially Mars.
It can be frustrating that consumers often have higher data rates than NASA, “but this has always been a fact of life for all space missions,” says David Israel, LCRD principal investigator at NASA’s Goddard Space Flight Center in Maryland. LCRD is a step toward making sure that future “communications and navigations capabilities are not constraints on the science and exploration missions,” he adds.
Hungry for bandwidth
What’s missing in all the planning for enormous satellite constellations and deep-space exploration is a commensurate increase in bandwidth to funnel data from space to scientists or business interests on Earth as well as software and commands out to the spacecraft.
The rise in demand is no longer theoretical. Consider, for example, NASA’s 9-year-old Curiosity rover and its frequent sampling stops to, among other tasks, scoop rocks and sand, and parse these samples for chemical details at a rate of 50 times per second. That sounds like lots of data until one looks at plans for the Mars Organic Molecule Analyzer from the German Max Planck Institute for Solar Systems Research set for launch in September 2022 on the European-Russian ExoMars rover. It will generate 50,000 data sets per second.
Showing how so much data can be transmitted through space and to the ground and vice versa will be the job of LCRD. While geosynchronous orbit is a small fraction of the distance to Mars, “characterizing and modeling the performance of the links through the Earth’s atmosphere and weather will apply to all Earth-space laser communications links,” Israel says by email. During the experiment, the space station and satellites in low-Earth orbit will be connected via laser with ground stations built in dry, mountainous areas to avoid the clouds that block optical transmissions. “Our experience operating the ground stations and flight terminals” — which contain the optical telescopes on the satellites — “will also directly apply, as well as the design, integration and test of space-qualified components.”
Testing technology
LCRD will be NASA’s most extensive optical communications demonstration since 2013, when the Lunar Laser Communications Demonstration transmitted high-definition video from lunar orbit to New Mexico at a rate of 622 megabits per second, about half as fast as what will be attempted with LCRD. Still, that was six times as fast as previous RF transmissions from the moon. But unlike the barely monthlong 2013 experiment, the LCRD tests will run for two years and possibly as long as five, giving researchers time to figure out whether optical communications could speed up delivery of imagery and telemetry from Earth orbit and establish internet connections between Earth and the International Space Station.
After a couple of months of in-orbit checkout by controllers at Goddard, NASA plans to spend two years experimenting with data transfer among Earth, geosynchronous orbit and low-Earth orbit. LCRD’s modems will encode data for optical modules that will generate the infrared laser beams. Those beams will be steered by lenses and mirrors with the aid of algorithms and a star tracker, the target being either a ground station or an optical communications receiver and transmitter scheduled to be mounted on the exterior of the International Space Station in 2022.
This optical payload will be tasked, for example, with relaying video, voice and text messages between the California and Hawaii ground stations. In addition, mission operators will experiment with coding and network protocols to figure out how to take full advantage of an orbiting optical communications node.
“LCRD is about to give us long-term operational experience to understand how to make the most of this optical technology,” says Israel, who is also the Goddard Exploration and Space Communications Projects Division architect.
With LCRD approaching its launch date, the civil and commercial space community in the United States and abroad has voiced interest in participating in the experiment. LCRD managers posted an invitation online for researchers to try out optical communications hardware and software with LCRD, including optical terminals that companies are developing for satellites and ground stations. Dozens of NASA researchers and people from other government agencies and companies have responded, including General Atomics Electromagnetic Systems, or GA-EMS, in Colorado, the company owned by General Atomics Corp. of San Diego that builds small satellites, laser weapons and more. GA-EMS wants to connect a commercial ground terminal with LCRD and to test various laser-pointing techniques.
“We certainly have no shortage of things we want to do, but there may be some overlap,” Israel says. “And people may propose things that we haven’t thought of.”
Until the 2013 Lunar Laser Communications Demonstration, some satellite communications experts questioned the suitability of laser communications for spaceflight. Would satellites be able to keep a narrow beam of light aimed directly at another optical device in space or on the ground? It turns out they can with the help of fast-steering mirrors and algorithms to stabilize and direct the light beam, but it’s not easy.
“When you add in vibration and the relative motion of the spacecraft, that becomes technically challenging,” Israel says.
Atmospheric turbulence and terrestrial weather further complicate matters. Turbulence scatters light waves, and clouds block them completely. To ensure optical beams have a clear path from geostationary orbit, NASA established one LCRD ground station at the Table Mountain Observatory in the San Gabriel Mountains above Los Angeles and another at Haleakala, a dormant volcano on the Hawaiian island Maui. In addition, NASA has developed models for predicting weather, which engineers hope to improve through LCRD experiments. “Between the pointing, acquisition and tracking challenges and dealing with the atmosphere and weather, that is where the cost comes in,” Israel says.
Flight terminals, meaning the optical telescopes, amplifiers and modems that spacecraft must have to send and receive science data or other information via laser, remain large and expensive. Each of LCRD’s telescopes will measure 10 centimeters. LCRD ground stations must be larger. The one in Maui is housed in a 5.5-meter-diameter dome.
Companies are developing optical terminals that will be as small as a flashlight to avoid taking up precious volume on a satellite and will be far less expensive. About 15 years ago, NASA budgeted about $90 million for the swiveling telescopes, computers and amplifiers that would transmit laser light from the car-size Mars Telecommunications Orbiter, decode the signals and forward the data. Laser terminal prices have fallen since then but high-reliability terminals like the ones NASA developed and integrated for LCRD with help from Northrop Grumman and the Massachusetts Institute of Technology Lincoln Laboratory, a federally funded research and development center, still can cost $15 million to $20 million apiece.
Driving down costs
Through the Blackjack program, DARPA hopes to drive costs down further, perhaps to “a couple hundred thousand dollars” each, Forbes says. “That will enable a larger number of satellites to take advantage of” optical signals to quickly send and receive data.
Optical equipment manufacturers call that price target ambitious but say the keys to reducing terminal prices are commercial vendors and mass production. “We’re able to figure out how to build things much more efficiently from top to bottom,” says David Czajkowski, founder, president and chief operating officer of Space Micro, a small San Diego company that builds spacecraft components. “We took a series of very reliable parts from data centers and found a way to qualify them for a 100-gigabit-per-second laser communication modem, which has been launched.” He says he’s not permitted to identify the customer.
GA-EMS, the General Atomics company, meanwhile, is also focused on reducing the cost of the optical terminals through high-volume production. “You don’t need a couple of these; you may need hundreds of these laser communication systems per year,” says GA-EMS President Scott Forney.
Under a $5.5 million contract from the Pentagon’s Space Development Agency, GA-EMS built two toaster-oven-size satellites with infrared cameras and optical terminals. The satellites, scheduled for launch in March, will gather and process infrared imagery day and night of objects on the ground and in the air, relay imagery back and forth, and transmit and receive data from optical terminals on the ground. In a future constellation with dozens or hundreds of satellites, what SDA calls a proliferated low-Earth orbit architecture, satellites and ground stations would have multiple pathways to transmit data directly among themselves and through optical terminals on aircraft, which is another technique to bypass clouds.
In addition to the promise of transferring video, voice and text messages between satellites as well as from space to the ground faster than RF, optical communications promise size, weight and power advantages. Optical signals stream from smaller telescopes backed up by smaller amplifiers in space and on the ground, compared with large RF dish antennas with powerful amplifiers.
The power savings comes from the laser focus, whether the optical signal is traveling from geosynchronous orbit or the moon. If it’s the moon, an optical beam might be a few hundred meters in diameter when it reaches Earth, as opposed to an RF beam that might be 500 or so kilometers in diameter.
“The primary advantage is that most of the power” — and therefore data — “gets to the receiver” in the optical terminal, says Tom Wood, senior director for optical communications and networking at CACI, a government contractor based in suburban Washington, D.C., that provides engineering services, IT support and mission support for U.S. and international government agencies.
CACI is building the bidirectional LCRD optical communications terminal for the space station under a NASA contract, optical terminals for NASA Orion crew capsules and a laser transmitter to send images and data directly to Earth from NASA’s Psyche spacecraft that in 2022 will be launched to the asteroid 16 Psyche. Because scientists think Psyche consists mainly of nickel and iron, like the cores of terrestrial planets including Earth, they want to inspect it in part to test their suspicion that it’s the exposed core of an early planet.
In addition to streaming data from deep space, satellite developers are eager to move data among satellites in Earth orbit because the technology can reduce the size, weight and power consumption of communications devices by 90% compared to RF, says Czajkowski, CEO of Space Micro, which manufactures RF and optical terminals.
Equally important for military customers is the enhanced security laser communications offer. In contrast to RF antennas, which transmit energy over a wide area, optical communications travel through a narrow beam, making them difficult to intercept or jam.
Imagine “looking down a soda straw,” Czajkowski says. “If someone shines a light from a different angle, it doesn’t interfere with light in the soda straw.”
In spite of all that promise, optical communications technology has yet to be widely deployed operationally, at least as far as the public record shows. The European Space Agency established the first optical link in 2001 between its Artemis geostationary telecommunications satellite and one of the French Spot Earth-observation satellites in low-Earth orbit. In 2001, the U.S. National Reconnaissance Office launched Geosynchronous Lightweight Technology Experiment or GeoLITE, a satellite equipped with an optical terminal to transmit data to the ground. NRO did not respond to requests for comment on whether its current fleet transmits data through optical links.
All told, advocates of optical technology want LCRD to be more than just the next in a long series of optical experiments. They want its launch later this year to mark a turning point year in the long history of this technology.
Related Topics
Communications satellitesIt can be frustrating that people in offices often have higher data rates than NASA, “but this has always been a fact of life for all space missions.” LCRD is a step toward making sure that future “communications and navigations capabilities are not constraints on the science and exploration missions.”
David Israel of NASA’s Goddard Space Flight Center
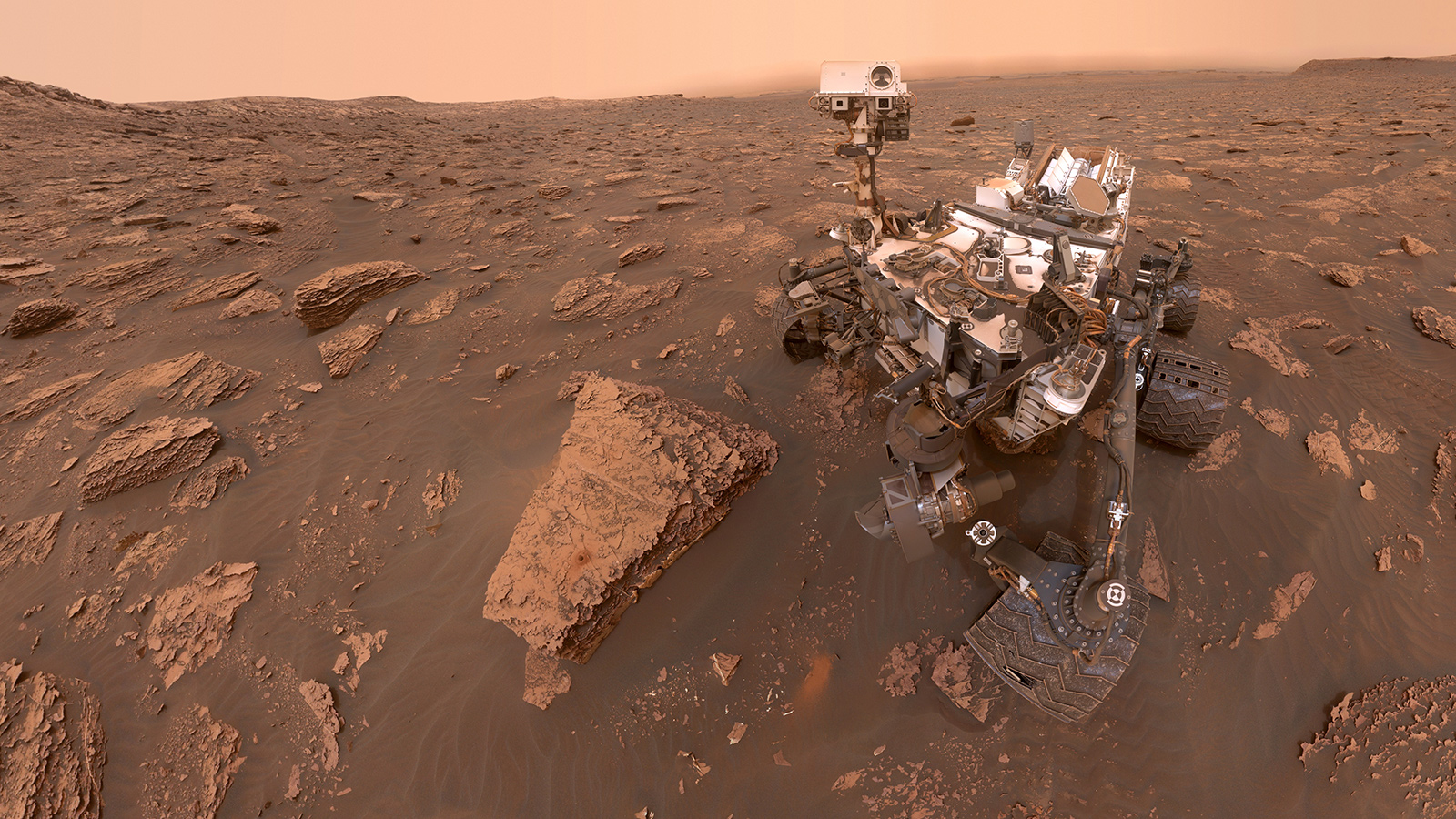
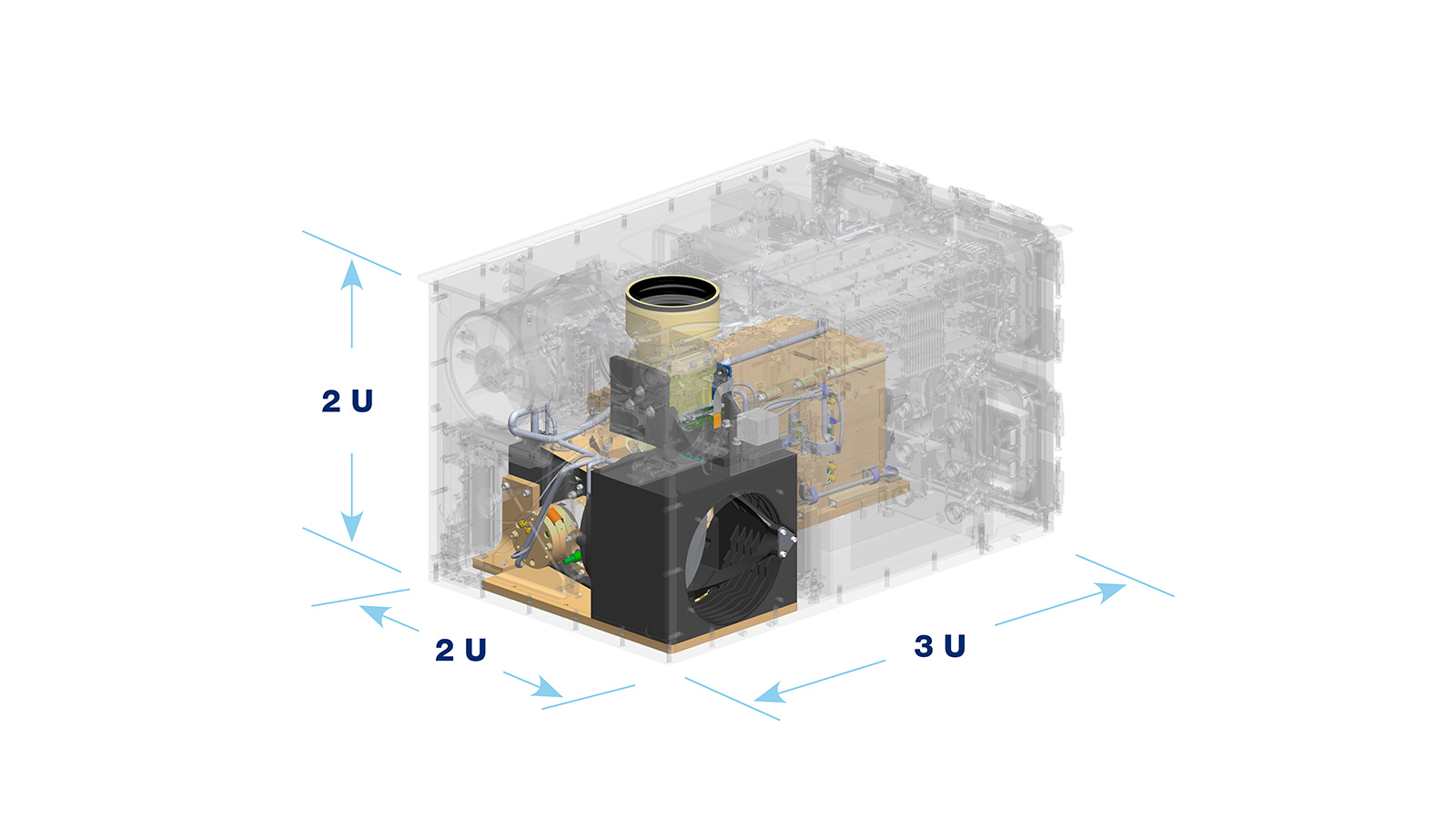
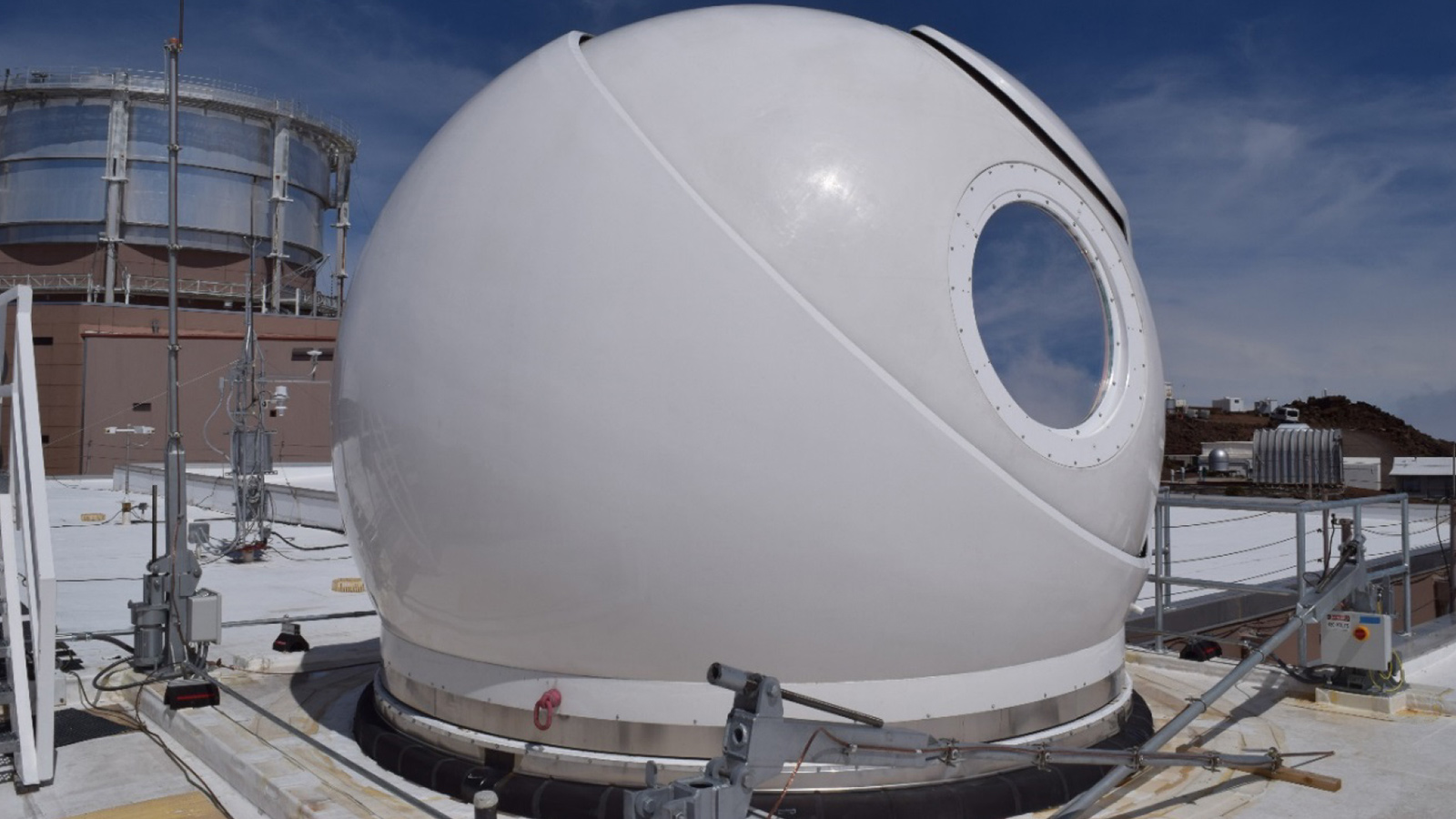