Green propellant
By Keith Button|March 2017
Experiment could move industry away from longtime propellant
At NASA Glenn Research Center’s combustion laboratory in Ohio, laser operators projected green-tinted light beams into a vacuum tank depressurized to simulate space. The lasers lit up the exhaust plume from a satellite thruster as high-speed digital cameras recorded the scene to map the chemicals in the plume.
These tests in 2014 of a new liquid propellant for satellites marked a turning point in a 30-year effort by the U.S. Air Force, NASA and the Department of Defense to wean the industry from thrusters powered by hydrazine liquid, an ammonia-smelling, toxic chemical. Among the problems with hydrazine is that it’s carcinogenic and is classified in the most hazardous category of propellants. Extensive precautions must be taken during production and pre-launch propellant loading.
In January 2016, Ball Aerospace of Colorado completed construction of the Green Propellant Infusion Mission, or GPIM, spacecraft that will test the newly conjured propellant and Aerojet Rocketdyne components designed to accommodate it. Engineers were anxious to start the mission this year, but the September launch on a SpaceX Falcon Heavy rocket will likely be delayed due to the backlog left by last year’s launch-pad explosion of a SpaceX rocket.
At the moment, GPIM is in storage in a Colorado cleanroom, but once in orbit it will fire its five thrusters repeatedly through a set of rigorous test objectives over the span of 13 months. The thrusters must point the spacecraft accurately; halt the spacecraft’s motion when controllers intentionally make it tumble; change its altitude as commanded; and operate even when the propellant tank is nearly empty or after being left idle for several months.
If all goes as planned, GPIM might convince the often risk-averse space industry to “infuse” the propellant and new components into future satellite thrusters as a hydrazine alternative.
Building the GPIM spacecraft was not as simple as loading different chemicals aboard. Engineers had to design and ground test new propellant tanks, fuel lines, filters and valves. Aerojet Rocketdyne devised a new kind of catalyst bed, which is the chamber in the thruster where the liquid propellant is turned into super-heated gas via chemical decomposition. The gas then expels from the chamber through the thruster’s nozzle, propelling the spacecraft in the direction the nozzle is pointed away from. At NASA Glenn, engineers measured the size and shape of the exhaust plume partly for GPIM but more so for designers of future satellites who must avoid accidentally directing the exhaust onto solar arrays and optics.
Hydrazine is familiar
In addition to its handling risks, hydrazine is quick to evaporate and difficult to store. Despite all that, hydrazine is the dominant propellant for orbiting spacecraft, says Christopher McLean of Ball Aerospace, who is the GPIM principal investigator.
The green fuel, designated AF-M315E, is a hydroxyl ammonium nitrate fuel/oxidizer blend. Advocates enjoy contrasting it to hydrazine. When loading hydrazine onto a satellite, workers must wear positive-pressure haz-mat suits. When handling AF-M315E, they need only lab coats, rubber gloves and splash visors. Drinking hydrazine would kill you but a bit of AF-M315E would be no more dangerous than ingesting an equivalent amount of isoniazid, a tuberculosis antibiotic. Hydrazine must be carried in explosion-proof tanks. The new liquid can be stored in plastic bottles and shipped via FedEx. Hydrazine fires are “incredibly nasty,” McLean says, but if the new propellant leaked onto a fire, it would put it out.
A 2012 Ph.D. dissertation by Christyl Johnson, now NASA Goddard’s deputy director for technology and research investments, found that the pre-launch processing and handling costs for the Swedish launch of a spacecraft powered by ammonium dinitramide, a green propellant that is more hazardous than the GPIM propellant, were $437,955 less than the equivalent costs for hydrazine. The U.S. Department of Transportation is reviewing proposed rules for the GPIM green fuel so that spacecraft builders can ship fully fueled, ready-to-launch green-propellant spacecraft across the country. The only step required at the launch site would be pressurizing the fuel tanks.
Perhaps the biggest advantage of the GPIM green fuel over hydrazine is its performance benefits. The green fuel is 45 percent more dense than hydrazine, meaning that a smaller volume of the fuel could substitute for hydrazine on a given spacecraft, and it generates 2 percent more thrust per pound of propellant, McLean says. Combined, the green fuel boosts performance by nearly 50 percent over hydrazine.
“In an industry where you’re always chasing that 1 or 2 percent better, 50 percent is pretty attractive,” says Julie Van Kleeck, vice president of advanced space development for Aerojet Rocketdyne, which designed and built the fuel tank, valves, lines, catalyst bed and thruster nozzles.
Solar-electric propulsion is another so-called “green” alternative for spacecraft and incredibly fuel efficient, generating 1,300 to 3,000 seconds of specific impulse, a measure of thruster efficiency, compared to 257 seconds for the green-fuel monopropellant and 235 seconds for hydrazine, McLean says. But because solar propulsion produces such minute amounts of thrust, it is better suited to geosynchronous-orbit and beyond-Earth-orbit spacecraft that can take weeks or months to perform a maneuver.
The main challenge for NASA and proponents of the green monopropellant fuel is to convince space mission planners that the alternative fuel and its thrusters, which have never flown in space, won’t fail. Hydrazine is well-understood, as are its effects on components.
“It’s very complex getting these new systems accepted on spacecraft, by the spacecraft user community, especially when you’re talking half-billion-dollar geocom assets and can we actually put new technology on them,” McLean says. Almost all of today’s commercial Earth-orbiting spacecraft are propelled by 1960s-era-thruster designs. New fuels have “a lot of inertia to overcome,” he says.
One key to acceptance is flight heritage: showing that a green-fuel-powered spacecraft has flown in orbit — starting with a single flight — without failing. “That gives us the ability to point back and say, ‘Hey, we’ve been through everything it takes to get this thing manufactured, integrated, processed, launched and operational,’” McLean says. “Every single one of those [steps] is a huge hurdle to overcome technically.”
Another key is providing data from the testing involved in the development steps to other spacecraft designers to make it easier for the designers to accept and incorporate green fuel propulsion in their own plans, says Matt Deans, a NASA co-investigator on GPIM.
“We have our technology valley of death, where you can develop a lot of new technologies on the ground, but when you go to fly them, you have to convince the mission planners to risk their multimillion-dollar instrument or a human life on a new technology, versus a technology that’s been proven in space many times,” Deans says.
When designers start digitally building their rough models for a new spacecraft, Deans says, one of the first steps is to map out where the thrusters will go — typically four pointing at 90 degrees to each other for attitude control — along with the thruster exhaust plumes — ice cream-cone shapes coming out of the thrusters representing “keep-out zones” for any part of the spacecraft that could be damaged by the chemicals, heat or forces in the exhaust.
The shapes and chemical makeup of hydrazine exhaust plumes are well-known, after decades of study, but the exhaust plume of the green propellant had never been characterized, Deans says. Liquid hydrazine breaks down easily through catalytic decomposition — passing over an iridium catalyst bed inside the thruster to create an exothermic reaction producing extreme heat with nitrogen and hydrogen gases, along with other small molecules, pushed out through a nozzle to generate thrust. The liquid green propellant also passes over an iridium catalyst to produce a similar chemical reaction, but with gasses with larger molecules, which can bounce off each other and push smaller molecules farther out to produce a wider-shaped plume. Also, water is one of the chief components of the green fuel’s exhaust, which could form ice on, or corrode, or cloud up a spacecraft surface within the exhaust plume.
“You have on many spacecraft very sensitive optics, and the last thing people want is water getting on their optics in the cold of space, because then you’re seeing ice,” Deans says.
Mapping exhaust plumes
At the Glenn Research Combustion Laboratory, NASA engineers tested the exhaust plume of a green-propellant 22-newton thruster built by Aerojet Rocketdyne to find out what chemicals were in the plume, at what concentrations and where in the plume they were. Mapping the plume was important to show the designers of future green-propellant spacecraft where the potentially corroding or icing chemicals were — those that could harm cameras or solar panels, for example, if the angle of the plume was miscalculated — as well as where forces or heat from the exhaust could bend or otherwise harm instruments on the satellite. Shining lasers of specific light frequencies through the normally clear exhaust plume, with high-speed digital cameras the engineers could identify types of molecules by the range of frequencies of energy the molecules gave off. They also marked the edges of the plume with Schlieren imaging, shining a bright light through the plume and recording the bending of the light passing through the plume with digital cameras. Then they checked the data against computer models, verifying the accuracy of the models and the ability of the computer models to predict other green-propellant plumes.
Deans says they discovered that the plume was generally wider than what a hydrazine plume would be from the same thruster, with the heavier molecules, like water and carbon dioxide, concentrated in the center of the plume and hydrogen on the outside, and with a tiny amount of hydrogen blowing back. At 10 centimeters away from the thruster, most of the exhaust’s heat had dissipated.
With the NASA exhaust plume test results, spacecraft designers can now plug the data into their own models for different thruster or nozzle sizes. “It’s a tool in everyone’s toolkit now,” Deans says. “We don’t want mission planners to start putting together their spacecraft and say: ‘I don’t know this; it’s too big of a risk for me.’ Now, it’s: ‘Oh, yeah: I have this nice little cone I can drop in my model.’”
“It’s one less thing for them to be concerned about when they build their missions,” Deans says. “We really wanted it so that when people go to plan this in future missions, nothing is really keeping them from saying: ‘I want to use this propellant.’”
Another difference between hydrazine and the green propellant are their flow characteristics: Hydrazine is like water; the green propellant is more like motor oil. Engineers at the NASA Goddard Space Flight Center built functioning table models of green-fuel delivery systems, complete with filters, valves and bended tubes — to assess the pressure and flow characteristics of each component. They also tested the propellant for slosh loads in tanks, which, for large volumes of liquid, could break tanks away from their moorings because of vibration during launch, or push a spacecraft slightly off course as the liquid in zero gravity resists moving in concert with the rest of the spacecraft, McLean says.
The Air Force Research Lab at Edwards Air Force Base in California, which invented the green propellant about 15 years ago, tested the green fuel to determine how much compression it could withstand before detonating.
“The Air Force tests it until it blows up, and then they say ‘OK, don’t exceed this pressure,’” McLean says. “Those guys have a lot of fun.”
NASA engineers at Goddard verified the Air Force lab’s pressure test results with “water hammer” tests and analysis. That term refers to the shock waves caused by fluid flow starting and stopping suddenly in fuel lines, such as when isolation valves are opened in space to allow the liquid propellant to flow into the lines connecting the tank to the thruster’s catalyst chamber for the first time, and then when the valves for the fuel lines are opened and closed during each firing of the thruster.
The GPIM spacecraft, a Ball BCP-100 cube satellite about 97 centimeters tall, will be dropped off at about 725 kilometers above the Earth, then fire its thrusters to drop to an orbit below 650 kilometers to ensure that it will slow down enough to fall back to Earth, and burn up, within 25 years. During its 13-month mission, the GPIM spacecraft will fire its thrusters during the first two months, then during one month in the middle of the mission and then for one month at the end, ending up at about 425 kilometers before shutting down. GPIM engineers will measure the health of the 1-newton thrusters in orbit by firing them during 200-millisecond impulses over various fuel pressures, then charting the rotational rates of the spacecraft to infer how much torque the firings generated.
Among all the engineering hurdles for the green propellant thruster, probably its biggest — a high combustion temperature — was cleared before the GPIM program started in 2012, McLean says. As with hydrazine, the green monopropellant exothermically decomposes — releasing intense heat — as it passes over a bed of iridium-coated ceramic pebbles in the catalyst chamber of the thruster. Hydrazine’s combustion temperature is 800 degrees Celsius; the green fuel’s combustion temperature is 1,800 degrees Celsius, and it needs to be heated before it will react with the catalyst.
Aerojet Rocketdyne developed a proprietary ceramic catalyst bed material, along with new materials for the body of the thruster’s reaction chamber, that could withstand the 1,000-degree temperature differences. Without the new ceramic material, the catalyst would melt and burn up in less than 10 seconds, McLean says, and GPIM wouldn’t have been possible. Aerojet Rocketdyne tested it, funded by the company and AFRL, before NASA started GPIM.
For material and process engineers, solving the extreme temperature problem and understanding its nuances was “either a nightmare or their best day, if they liked challenges,” says Van Kleeck of Aerojet. “It’s an engineering problem; it’s not that you have to have brand-new science. But it’s not an easy engineering problem to solve.”
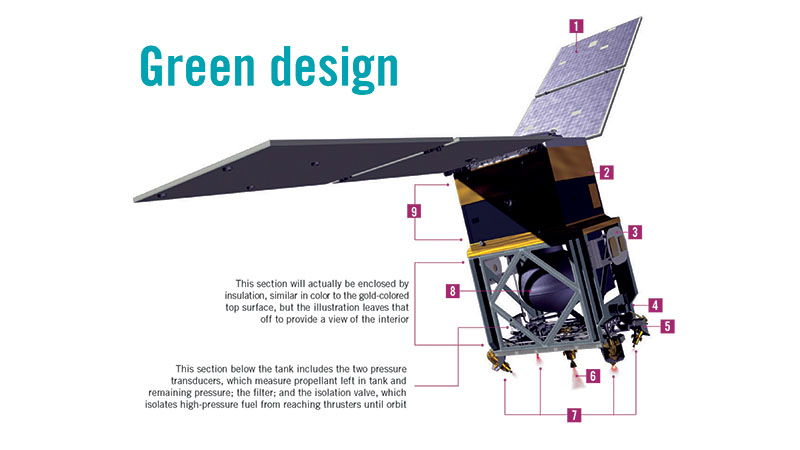

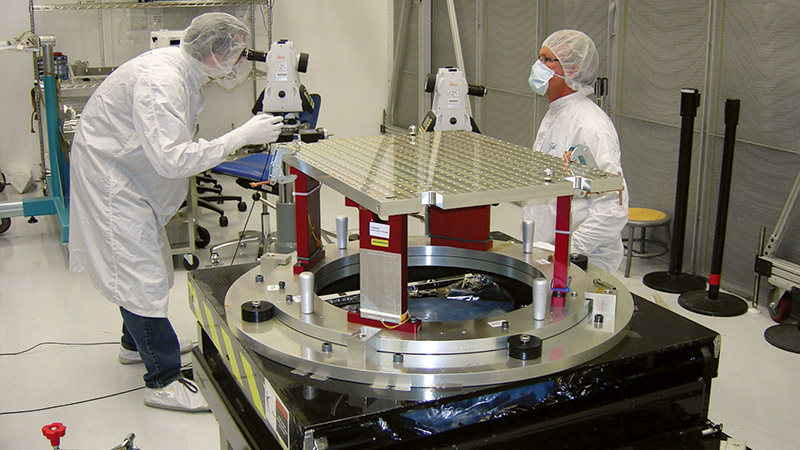