Cosmic GPS
By Adam Hadhazy|May 2020
The U.S. Global Positioning System has changed how we operate spacecraft in low-Earth orbit. Now GPS is starting to do the equivalent for spacecraft flying beyond the GPS constellation, and someday possibly all the way to the moon, where positioning contributions from other nations could add up to stunning accuracy. Adam Hadhazy tells the story.
Before GPS receivers became standard issue on low-Earth orbit satellites, the spacecraft had limited information on where they were and where they were going. Control stations on the ground needed minutes or even hours to process radar signals bounced off of these LEO satellites to calculate position and velocity and then send that info back up to orbit. The advent of GPS changed the game, making precision navigation and timing information on board the satellites available in real time. The system provides key time-tagging for communications, Earth observations and other satellite services, while keeping those services available nearly 24/7 and slashing fuel use because satellites no longer have to be taken offline for large, station-keeping thruster burns.
For LEO spacecraft whizzing underneath the GPS constellation’s altitude of 20,200 kilometers, tuning into GPS was a straightforward affair of turning their receivers upward to collect the readily available rain of GPS signals pouring down toward Earth. Twenty years ago, researchers proved that this signal collection could actually be done above the GPS constellation. The trick was to feed on GPS scraps, as it were, by capturing the weak “spillover” signals from GPS satellites on the far side of Earth that beam right past the planet, missing their terrestrial target. The breakthrough of snagging this spillover led to the adoption of GPS aboard satellites in geostationary orbit, some 36,000 kilometers above the equator, and to demonstrating the capability as far out as halfway to the moon.
Now, for their next feat, researchers want to equip new spacecraft to benefit from GPS all the way at the moon and even down to its cratered surface. If successful, this capability would go a long way toward enhancing operations in what could one day be a very busy lunar environment.
“It’s really only been since late 2018, early 2019 where we could show that lunar was feasible,” says engineer Frank Bauer, a 30-year NASA veteran and now president of FBauer Aerospace Consulting Services in Maryland.
“What GPS has done for spacecraft in LEO has been phenomenal,” says Bauer. Likewise, “GPS could be transformative for lunar missions.”
Future endeavors could harness GPS to help build out a durable human presence in cislunar space between Earth and the moon. Envisioned enhancements include boosting autonomous spacecraft operation with real-time navigation and precise timing for formation flying, rendezvous and docking, station keeping, and more.
Extending the reach of GPS
For Bauer and others, getting to this point has been a two-decade personal quest.
Developed in the 1970s and then made available for civilian use in the 1980s, the U.S. Air Force-operated GPS constellation service was first demonstrated out to low-Earth orbit in 1982 by the Landsat-4 Earth-imaging satellite.
Bauer and colleagues at NASA’s Goddard Space Flight Center in Maryland realized that it might be possible to install GPS equipment on new satellites destined for duty out past the GPS constellation. These beyond-the-constellation GPS receivers would have to capture those spillover signals that spread from each GPS satellite along multiple radio beam pathways, called lobes. The main lobe of a GPS radio signal beam’s width forms a V-shape. While most of the main lobe hits Earth as intended, some of that “V” extends beyond the limb, or curve of the planet, and into empty space. Residual signal coming out of the transmitting antenna forms side lobes that pass well clear of Earth. Normally, engineers disregard side lobes as wasted energy. The side lobes are quite weak — only about 3% of the total signal strength, whereas the main lobe constitutes 97%. But so long as there is line of sight to these side lobes, and better yet a bit of spillover from the main lobe, a sufficiently sensitive receiver aboard a spacecraft on the opposite side of Earth from the GPS-transmitting satellite can detect the signal.
The initial big step for proving out this capability came when Bauer and colleagues piggybacked a GPS receiver on a satellite called OSCAR-40, short for the Orbiting Satellite Carrying Amateur Radio. The satellite was launched in 2000 for the Radio Amateur Satellite Corp., or AMSAT, where Bauer continues to serve as the vice president of human spaceflight programs. OSCAR-40 ultimately reached a maximum altitude of about 60,000 km and detected GPS signals at this rarefied height, marking the first-ever demonstration of GPS in this new regime.
The proof of principle was now in place. Next, creating reliable GPS beyond the constellation required the engineers to deal next with weak signals and situations in which few satellites are available. On Earth, receiving signals from three GPS satellites produces a rough position, and a fourth delivers accuracy to within meters. Getting out past the GPS constellation, though, satellite availability (through signal line of sight) can be poor because Earth tends to get in the way. So for those times when only a single GPS satellite is in view, the Goddard team developed a software package, dubbed GEONS (GPS-Enhanced On-board Navigation System). GEONS accepts a single GPS signal, combining that information with other onboard sensors, such as the accelerometers and gyroscopes in an inertial navigation system, to arrive at precise time and position solutions.
As for dealing with signal weakness, a terrestrial analog is the all-too-familiar problem of straying too far from a cell tower to maintain a strong connection. “The farther out you go, the poorer reception you have, so you’re going to have to augment what the receivers are capable of,” says Joel Parker, the positioning navigation and timing policy lead at Goddard, who joined NASA shortly before Bauer’s retirement from the agency in 2011 and whom Bauer later mentored for the role. “The real magic of this is to pick up signals that are really weak because of the losses that are inherent to that big of a distance.”
A mission selected for development by NASA five years after the OSCAR-40 breakthrough provided the vehicle to test out the GEONS software and the sensitive receivers and at far greater altitudes. The Magnetospheric Multiscale, or MMS, mission called for sending four octagonal satellites into space to measure the transfer of energy between the sun’s magnetic field and Earth’s for space weather forecasters. The MMS satellites would need to fly in a tight tetrahedral formation to measure the structure of these magnetic fields in three dimensions. GPS or a technology with its capabilities was mission-critical. “You can’t do formation flying unless you really know where you are continuously,” says Bauer, who had a long-standing interest in formation flying because of the exquisite navigation precision it requires. He and his colleagues quickly became involved in discussions with MMS engineers on the project.
Development work culminated in the Navigator GPS Receiver, which they loaded with the GEONS software and flew on each 3.5-meter-wide MMS satellite when they were launched together in 2015. The receivers proved highly capable, even at apogee on an elliptical orbit extending to 190,000 km, or about halfway to the moon. Today, the MMS spacecraft continue to fly synchronously with just 7.2 km separating them — the closest formation flying for spacecraft ever demonstrated, NASA says. “We’ve broken world records now with MMS,” says Bauer.
Precision through stability
As MMS was going through its early paces in orbit, the GOES-R weather satellite, the first of NOAA’s four next-generation Geostationary Operational Environmental Satellites, was launched in November 2016. The satellite (rechristened GOES-16 once aloft) became NOAA’s first geosynchronous satellite to be equipped with GPS. The satellite, now in the GOES-East slot for an optimal view of the Atlantic Ocean and Caribbean and Eastern side of the Americas, was built by Lockheed Martin under NASA’s management at Goddard.
The GOES program had originally provided funding for the OSCAR-40 experiment, recognizing the potential that GPS could have for precision weather forecasting. Bauer had been involved here as well, serving as an architect for the control system of the predecessors to the newest satellites.
In February 2019, the similarly equipped GOES-17 was declared operational in the GOES-West slot for a view of the Americas and the Eastern Pacific Ocean. Prior GOES generations had to perform station- keeping maneuvers every few weeks, taking the satellites offline for several hours at a time. Now, both GOES-16 and GOES-17 keep on obtaining multispectral images while performing minuscule thruster burns for station keeping with very minimal downtime.
“GPS navigation helps keep the spacecraft rock steady in terms of where it thinks it is,” says Parker.
GOES-16 and 17 keep a constant watch on tropical cyclones and other weather systems as they evolve, ultimately gathering four times the spatial resolution of prior GOES generations. “It’s like going from a standard-definition screenshot” with the other satellites “to a full-motion, high-definition movie” with the newest satellites, says Parker.
Bauer says the onboard GPS delivered even better stability than expected. “I would have been happy to get 100-meter performance at GEO,” says Bauer, “but what we have is like what you’ve got on the ground.” On Earth’s surface, GPS is typically accurate to within 5 meters, indeed matching the low end of one type range error with of the newest satellites.
Surprisingly to those who pioneered the technology, despite the success on GOES-16, GPS utilization remains scant among the several hundred satellites at GEO, says Parker. That said, companies such as Virginia-based General Dynamics (which built GOES-16’s receiver) are now offering commercial receivers for this orbital region, having licensed the NASA technology originally developed for Navigator.
James “JJ” Miller, deputy director of policy and strategic communications within the Space Communications and Navigation Program at NASA headquarters, hopes more companies and government program offices choose to adopt GPS beyond the GPS constellation, because better positioning could multiply the number of spacecraft able to safely operate in the increasingly congested GEO — just like how GPS has enabled commercial aircraft to fly closer together.
“If you had every GEO bird in the future equipped with GPS,” says Miller, “you could in essence create real estate in the space domain because you could reduce the separation distance between spacecraft.”
Realizing lunar GPS
The next step would be to extend GPS where humans haven’t trodden in nearly half a century.
In simulations run in 2018, based in large part on MMS and GOES-16 data, Bauer, Parker and colleagues worked out the number of GPS satellites that would be visible in the near-moon environment and what sort of signal strengths could be expected. The upshot: With relatively modest upgrades to existing post-constellation GPS equipment, lunar GPS appears eminently possible.
A more powerful receiving antenna will be required, perhaps with about 14 decibels of gain, a measure of the amount of power transmitted in a particular direction compared to an antenna radiating equally in all directions. Another element: including actuators to keep the antenna pointed at Earth to maximize line of sight, because the Earth-orbiting GPS constellation spans less than 10 degrees on the lunar sky. (For comparison, when viewed from Earth’s surface, the moon is half a degree in diameter.)
So far, so simple, and based on components already routinely incorporated into spacecraft. “It’s not cost-intensive,” adds Parker. The one pricey bit of equipment would be including atomic clocks, as are found in GPS satellites, aboard a lunar-bound, GPS-signal-receiving spacecraft. That second clock or clocks would be good for extremely precise timekeeping on the spacecraft’s end, which further cuts down on overall error. But the ultraprecise ticks from a single such clock — for instance, if installed on NASA’S proposed lunar Gateway station under NASA’s Artemis program — could be disseminated and received by any space vehicles in radio vicinity, Parker says.
Toward these ends is the currently deployed state-of-the-art, deep-space GPS unit, called NavCube, developed by colleagues of Parker’s at Goddard. NavCube is a 25-by-20-by-15 centimeter unit that weighs about 5 kilograms and is attached to the exterior of the International Space Station on the P3 truss segment. The device is part of a broader experiment to test out the feasibility of ultra-high-data X-ray communications, which would require precise timing, and is also expanding GPS capabilities in space. Unlike its predecessor, Navigator, flown on MMS, and which only received a single GPS frequency, NavCube receives both L1 and L2 signals, with L2 being a more accurate civil signal now available from 19 of the GPS constellation’s satellites.
(L3 is reserved for transmitting data on detected nuclear explosions, and L4 is not yet in service.) Continuing work on the ground at Goddard is looking into use of L5 signals, which would be twice as powerful and have wider beam width than L1 and L2 signals, and thus would provide increased visibility out at the moon.
Even more help could be on the way. Bauer, Parker, Miller and others are all working through the United Nations International Committee on Global Navigation Satellite Systems to foster interoperability among all six major GNSS, short for Global Navigation Satellite Systems. Besides GPS, the five other constellations are the European Union’s Galileo, Russia’s GLONASS, China’s BeiDou Navigation Satellite System, India’s NavIC and Japan’s Quasi-Zenith Satellite System. Adding in those constellations would triple the number of available satellites from the U.S. fleet of 27 (plus four on-orbit spares) to circa 100.
If it were possible to collect everyone’s GNSS signals, Bauer says accuracy at the moon would be on the order of 1 km — certainly useful. But if atomic-clock precision were incorporated into the lunar-bound spacecraft, or fed to it by the Gateway, for instance, the accuracy could improve to more like 100 meters. That accuracy should be sufficient to enable efficient, autonomous navigation through cislunar space toward the Gateway — the driver, the iron and the chip shot. The short putt or docking would then be handled by conventional means of radar, lidar and cameras. Excursions to the lunar surface could likewise count on GPS for most of the descent. “You probably switch from using GPS almost exclusively to lidar and then visual sensors to make sure you don’t hit any boulders or whatever as you come in for landing,” says Bauer.
Getting your bearings, at terra and luna
The first full demonstration of lunar GPS could come as soon as mid- to late 2021, when a Space Launch System rocket lifts off from Florida carrying an uncrewed Orion crew capsule on the Artemis-1 mission. Orion will orbit the moon for several days, capping a three-week deep-space jaunt. Orion will keep its GPS receivers on during both the outbound and inbound legs in order to characterize how much useful GPS signal is available.
For researchers who have long wanted precision positioning in deep space, being able to take the existing system with us, so to speak, versus having to construct a new system, would be welcome news. “Ten years ago, people talked about building another GPS constellation around the moon,” says Parker, the policy lead at Goddard. “Now we know we can use what already exists.”
Still, that build-out possibility has not been abandoned, given the expected limited precision of relying on GPS spillover from Earth, and in light of the advent of small satellites. One NASA concept, dubbed LunaNet, would consist of small sats that would augment terrestrial position and timing, while also routing communications among astronauts, explorers, astrophysicists or prospectors.
The more data brought to bear for position, velocity and time, the better, says Bauer. “Even ancient navigators fused navigation sensor data,” he says, “using a compass, chronometer and a sextant or the stars and sun to navigate the oceans.”
If the most ambitious plans for lunar activity next decade come to fruition, cislunar space could become just the latest ocean for our species to traverse. “We want to establish a sustainable human presence at the moon,” says NASA’s Miller. “There’s a lot of things we could do there in our lifetimes.”
Related Topics
Space Science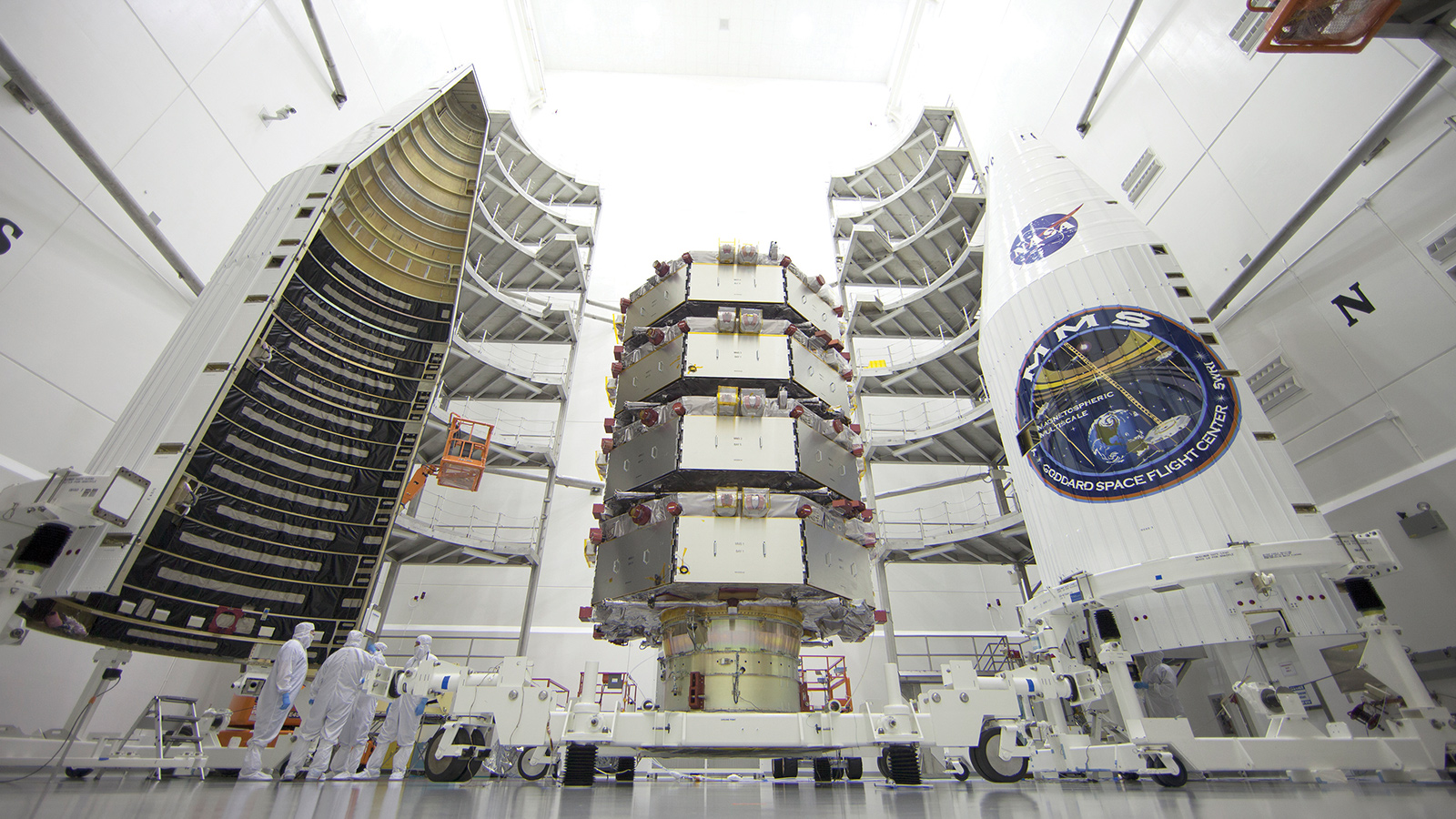
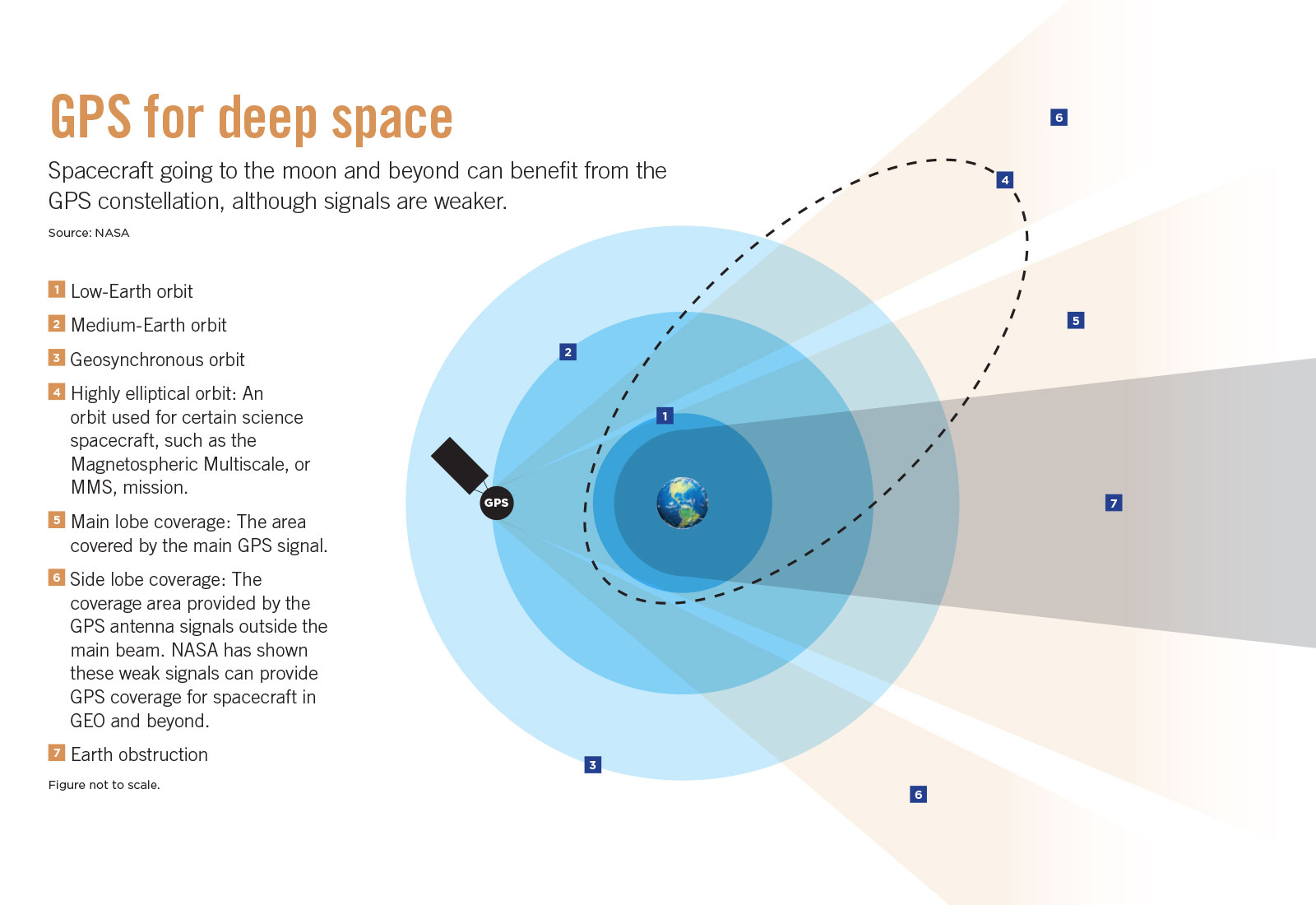
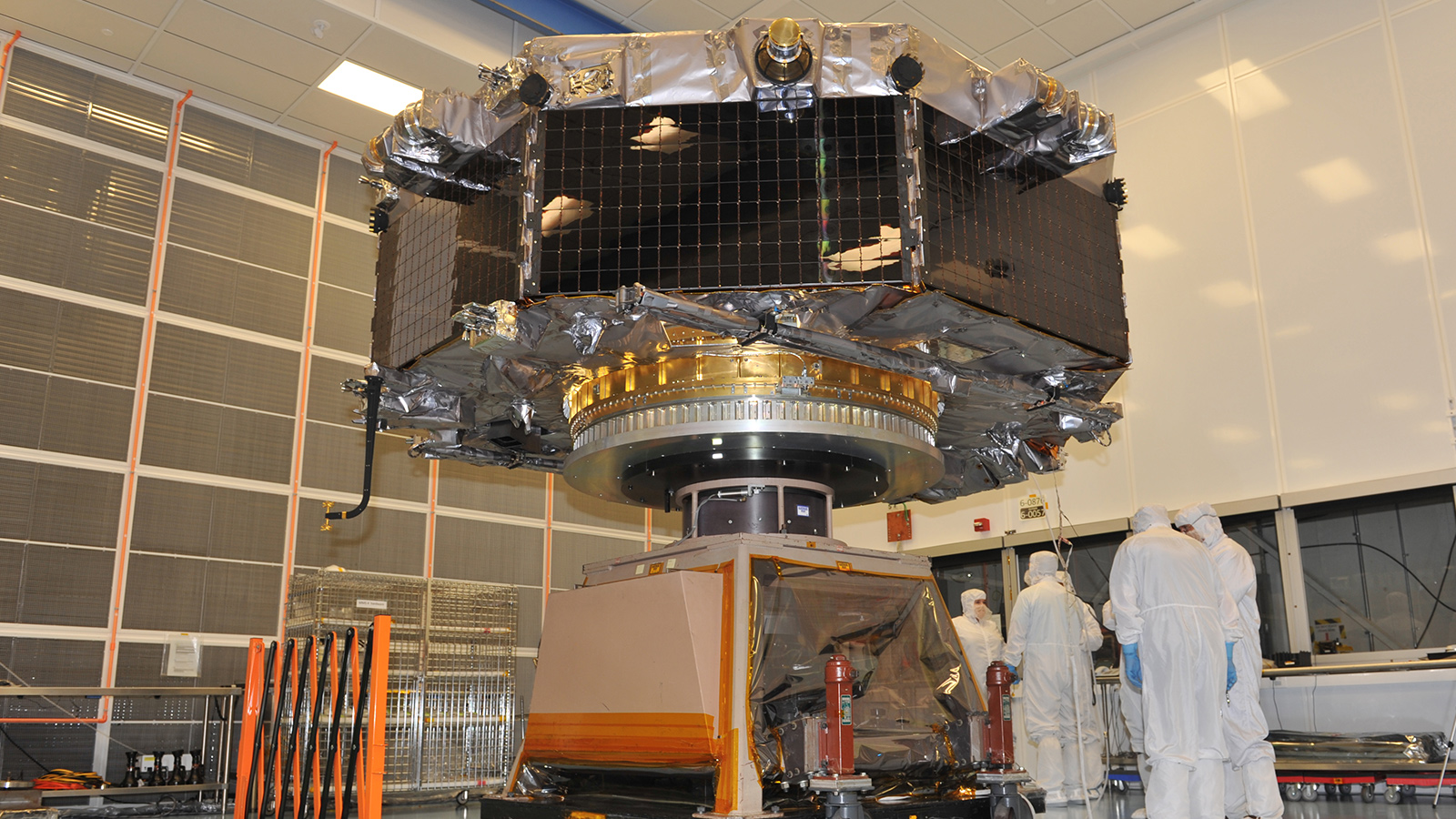
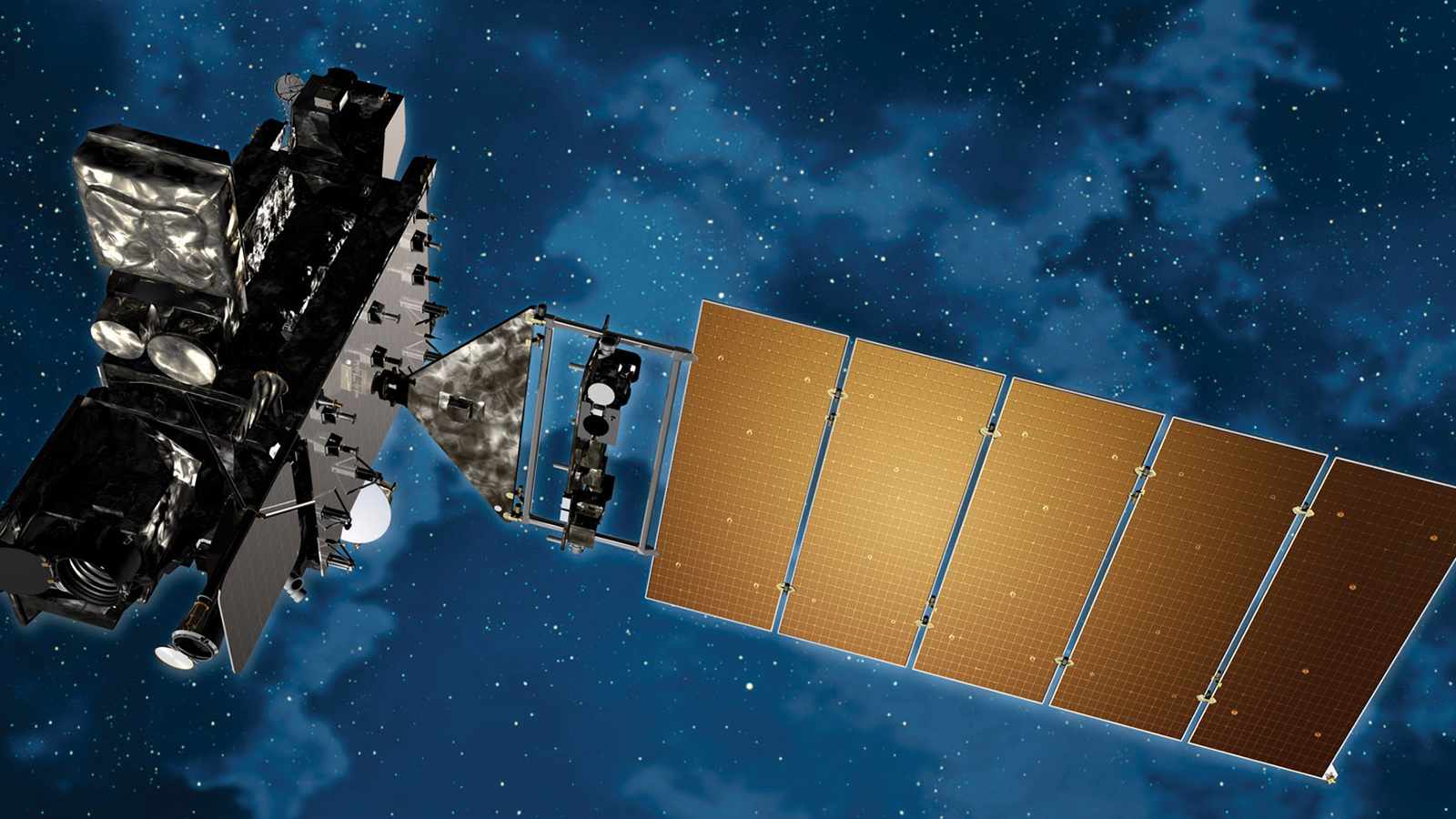