Blackout busters
By Keith Button|April 2017
Engineers to test a solution to re-entry communication problem
Aerospace engineers in Germany watched through cameras as they activated a superconducting magnet to push aside electrons in a plasma layer surrounding the surface of a simulated space capsule in a vacuum chamber.
They hoped to form a narrow channel in the plasma through which radio signals could travel.
The engineers in these May 2015 tests were trying out a possible solution for the radio blackouts that have plagued returning space capsules since the 1960s. The heat and pressure generated as a capsule plows into the atmosphere at 7 to 9 kilometers per second break electrons away from molecules of oxygen and nitrogen. The resulting plasma blocks transmission and reception of radio signals for up to several minutes.
These tests and others at DLR, the German Aerospace Center, could ultimately point the way to solving the blackout problem. If so, the scientists and engineers have their work cut out. The technique tested in 2015 could prove difficult to convert into a functional blackout-busting tool, and the results of those tests were mixed. The team hopes for better results when the alternative Stanford University technology is tested at DLR sometime after July 1.
The first challenge the engineers faced for the 2015 tests was devising a setup that could accurately mimic the extreme plasma conditions of a spacecraft returning to Earth. The engineers chose argon, because it’s easier to turn into plasma than the atmospheric gases a spacecraft would encounter. Argon turns to plasma at about 3,700 degrees Celsius (6,700 Fahrenheit), versus about 6,700 C (12,000 F) for normal air, says Ali Guelhan, head of the DLR’s supersonic and hypersonic technology department.
Even at these lower temperatures, the engineers must be careful not to burn up the electrodes that create the electric arc that heats the argon. They achieved that by rotating the position of the arc with magnets, so that the heat was never in one spot on the electrodes long enough to damage them.
The argon was compressed into plasma in a high-pressure chamber and then spewed through a nozzle into the vacuum chamber where the capsule was located. The pressure differential accelerated the plasma to a hypersonic speed of about 2,000 meters per second.
One task in the tests was to define the characteristics of the plasma — the density of its electrons, for example — that determined the point at which radio signals could get through, says Lars Steffens, a DLR engineer who worked with Guelhan on the blackout tests. In general, transmitting on higher radio frequencies gave the signal a better chance of getting through the plasma. Penetrating the plasma was more and more difficult as the density of electrons in the plasma grew.
The argon nozzle produced a shroud of plasma that was 20 to 30 centimeters wide and 10 centimeters thick. The radio transmitter inside the capsule produced signals that exited the capsule through a radio-wave-friendly quartz surface — not the copper nose — to reach a receiver behind the plasma and the nozzle.
The engineers manipulated the argon plasma with superconducting magnets mounted inside the capsule, producing a magnetic field with a strength of up to 1.5 teslas, which cleared a path through the plasma electrons for certain high-frequency signals to make it through. The effect was not as great as the engineers initially believed it would be, Guelhan says.
Keeping the superconductor magnets at the right temperature was a challenge. The magnets had to remain at temperatures below minus 265 degrees C while the super-hot plasma flowed just 1 centimeter away, along the surface of the capsule. That cooling issue, along with their weight, would make it extremely difficult to carry the magnets aboard a space vehicle, Guelhan says.
Sometime after July 1, Guelhan and Steffens will apply this test setup to an alternative concept — pulsed electrostatic manipulation — developed by Siddharth Krishnamoorthy, an aerospace engineer at Stanford University studying the blackout issue. Krishnamoorthy wants to zap an electrode just under the surface of a space vehicle with short bursts of electricity to push electrons in the plasma aside, similar to the magnet concept, to create a path for the radio signal to get through.
Krishnamoorthy says he decided to study an electric pulse solution to the radio blackout problem because it seemed more promising than other possible solutions, such as shaping spacecraft to produce thin areas of plasma during entry.
Radio blackout wasn’t a problem for returning space shuttle orbiters, once NASA could relay radio signals to the ground through the orbiting Tracking and Data Relay Satellite System spacecraft. The shape of the orbiters created an area on top, facing away from Earth, where the plasma’s density was low enough for radio signals to get through, Krishnamoorthy says. It is possible for engineers to design specially shaped spacecraft that can create low-density areas of plasma during re-entry, like the space shuttle did, so radio signals could pass through. One problem with that idea is that the design can’t adapt to changing flight conditions that create different plasma issues.
“If your flow conditions change, or if your trajectory changes, you would have to go through the entire design process again and come up with a new shape,” Krishnamoorthy says. “So it’s not very agile in terms of shifting conditions, and it turns out that hypersonic flow actually is very noisy and very shifting.”
Another problem with the aerodynamic shaping solution for re-entry blackout is that the designs produce sharp edges, which are not desired for re-entry vehicles because they transfer more heat to the spacecraft.
Krishnamoorthy is focusing on capsule vehicles, like Orion, which he calls the “go-to shape for most re-entry vehicles,” now that NASA has retired the shuttle fleet. It is the electrons in the plasma that are blocking the radio signal, because of their increased density in the plasma, Krishnamoorthy says. The electrons are so light, and have such little inertia, that when the radio signal — an electro-magnetic wave — attempts to pass through, the electrons just cancel it out.
An electrode inside the capsule will apply an electric field to the plasma to repel electrons from the antenna region. That electric field also attracts ions, but because the ions are much heavier than the electrons, they are attracted much slower than the electrons are rejected, and a path is cleared for the radio signal.
A magnetic field also can accomplish the electron movement, but integrating a magnetic field on a spacecraft would be complicated, he says. “My guiding principal was let’s try to investigate what it takes to do this if you just use an electric field, and no magnetic field.”
Krishnamoorthy knew that electric fields could also pose safety problems for spacecraft, so he came up with the idea for pulsed electric fields. “Leaving high electric fields on all the time on a spacecraft is very dangerous, because it can cause arcs, and sparking and shorting,” he explains.
The Stanford professor has been running computer simulations of the pulsed electrostatic manipulation to help define the specific characteristics of the electrode, insulation, voltage level and other factors to employ in the DLR chamber testing. The computer testing model is based on particle-in-cell simulation of the plasma, involving hundreds of millions of particles and a large digital grid to account for them. Each simulation of the testing run on Stanford’s computing network — taking up the equivalent of about 500 central processing units — takes from a few hours to more than a day, in some cases.
Based on the simulations, applying a higher voltage clears a larger area for the radio signals to get through, as does applying the pulses faster, Krishnamoorthy says. The reduction is enough to let L-band and S-band radio signals through.
DLR’s Guelhan says high voltage testing of Krishnamoorthy’s approach presents some challenges for the upcoming tests. Up to 10 kilovolts of short, 0.01- to 10-microsecond bursts must be generated, but the researchers must block those sparks from jumping to surfaces they aren’t intended for. “But,” he says, “It’s probably much easier to achieve than the cooling of the magnets.”
Related Topics
Human Spaceflight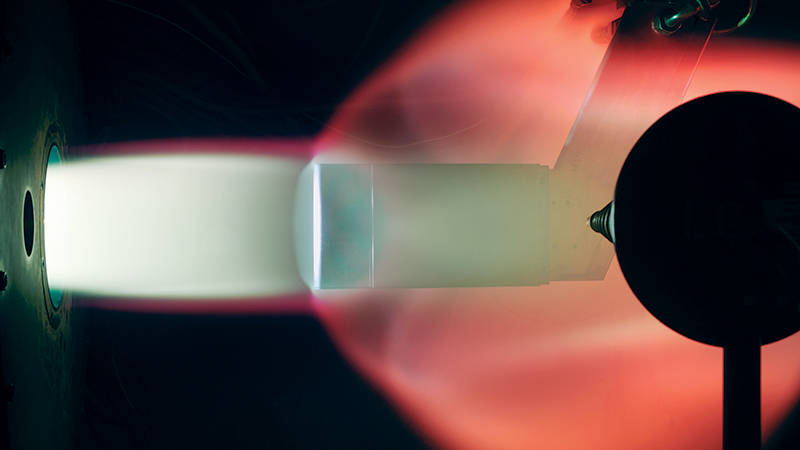
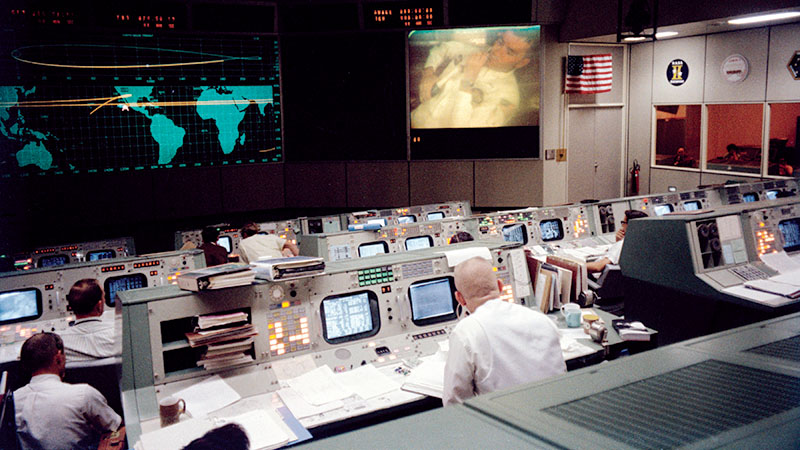